ISS Research Program
NASA’s Physical Sciences Research Program at the International Space Station (ISS) has conducted striking fundamental and applied research leading to improved space systems and new, advantageous products on Earth.

International Space Station Environments, Power and Research
NASA’s experiments in the various disciplines of physical science, reveal how physical systems respond to the near absence of gravity. They also reveal how other forces that on Earth are small compared to gravity, can dominate system behavior in space. The International Space Station (ISS) is an orbiting laboratory that provides an ideal facility to conduct long-duration experiments in the near absence of gravity and allows continuous and interactive research similar to Earth-based laboratories. This enables scientists to pursue innovations and discoveries not currently achievable by other means. NASA’s Physical Sciences Research Program also benefits from collaborations with several of the ISS international partners—Europe, Russia, Japan, and Canada—and foreign governments with space programs, such as France, Germany and Italy. The scale of this research enterprise promises new possibilities in the physical sciences, some of which are already being realized both in the form of innovations for space exploration and in new ways to improve the quality of life on Earth.
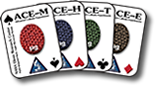
Grid View List View
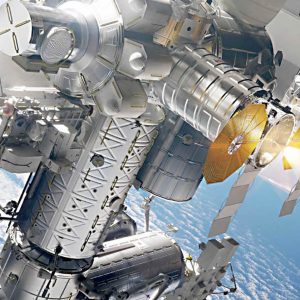
1 (lowest) | 2 | 3 | 4 | 5 (highest) |
---|---|---|---|---|
1 (lowest) | 2 | 3 | 4 | 5 (highest) |
---|---|---|---|---|
Thanks for contacting us! We will get in touch with you shortly.
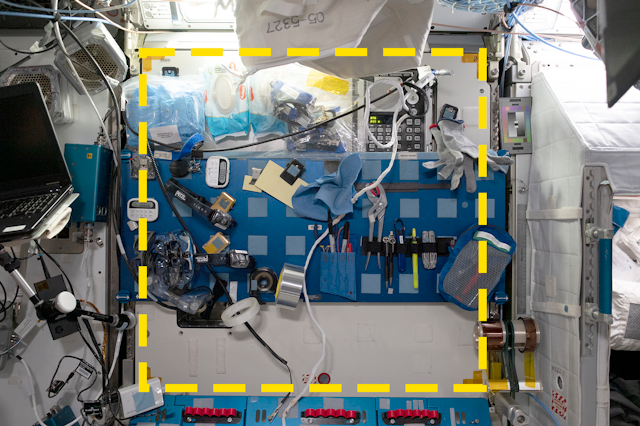
Archaeologists conduct first ‘space excavation’ on International Space Station – and discover surprising quirks of zero-gravity life
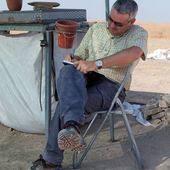
Professor of art history, archaeology, and space studies, Chapman University
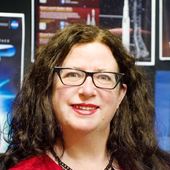
Associate Professor in Archaeology and Space Studies, Flinders University
Disclosure statement
The SQuARE experiment was sponsored by the ISS National Laboratory and funded by Chapman University. Axiom Space was the Implementation Partner.
Alice Gorman has previously received funding from the Australian Research Council.
Flinders University provides funding as a member of The Conversation AU.
View all partners
New results from the first archaeological fieldwork conducted in space show the International Space Station is a rich cultural landscape where crew create their own “gravity” to replace Earth’s, and adapt module spaces to suit their needs.
Archaeology is usually thought of as the study of the distant past, but it’s ideally suited for revealing how people adapt to long-duration spaceflight.
In the SQuARE experiment described in our new paper in PLOS ONE, we re-imagined a standard archaeological method for use in space, and got astronauts to carry it out for us.
Archaeology … in … spaaaaace!
The International Space Station is the first permanent human settlement in space. Close to 280 people have visited it in the past 23 years.
Our team has studied displays of photos, religious icons and artworks made by crew members from different countries, observed the cargo that is returned to Earth, and used NASA’s historic photo archive to examine the relationships between crew members who serve together.
We’ve also studied the simple technologies, such as Velcro and resealable plastic bags, which astronauts use to recreate the Earthly effect of gravity in the microgravity environment – to keep things where you left them, so they don’t float away.
Most recently, we collected data about how crew used objects inside the space station by adapting one of the most traditional archaeological techniques, the “shovel test pit”.
On Earth, after an archaeological site has been identified, a grid of one-metre squares is laid out, and some of these are excavated as “test pits”. These samples give a sense of the site as a whole.
In January 2022, we asked the space station crew to lay out five roughly square sample areas. We chose the square locations to encompass zones of work, science, exercise and leisure. The crew also selected a sixth area based on their own idea of what might be interesting to observe. Our study was sponsored by the International Space Station National Laboratory.
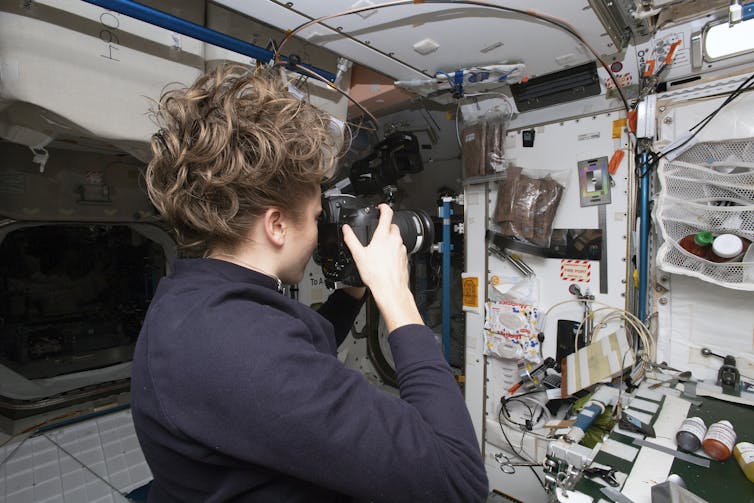
Then, for 60 days, the crew photographed each square every day to document the objects within its boundaries. Everything in space culture has an acronym, so we called this activity the Sampling Quadrangle Assemblages Research Experiment, or SQuARE.
The resulting photos show the richness of the space station’s cultural landscape, while also revealing how far life in space is from images of sci-fi imagination.
The space station is cluttered and chaotic, cramped and dirty. There are no boundaries between where the crew works and where they rest. There is little to no privacy. There isn’t even a shower.
What we saw in the squares
Now we can present results from the analysis of the first two squares. One was located in the US Node 2 module, where there are four crew berths, and connections to the European and Japanese labs. Visiting spacecraft often dock here. Our target was a wall where the Maintenance Work Area, or MWA, is located. There’s a blue metal panel with 40 velcro squares on it, and a table below for fixing equipment or doing experiments.
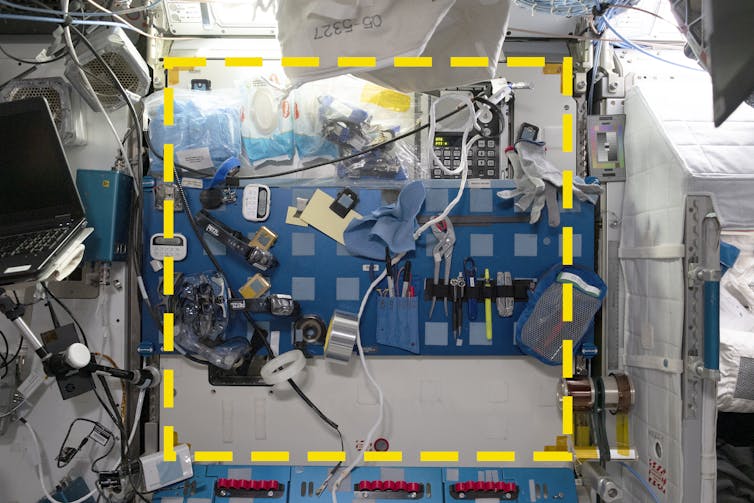
NASA intended the area to be used for maintenance. However, we saw hardly any evidence of maintenance there, and only a handful of science activities. In fact, for 50 of the 60 days covered by our survey, the square was only used for storing items, which may not even have been used there.
The amount of velcro here made it a perfect location for ad hoc storage. Close to half of all items recorded (44%) were related to holding other items in place.
The other square we’ve completed was in the US Node 3 module, where there are exercise machines and the toilet. It’s also a passageway to the crew’s favourite part of the space station, the seven-sided cupola window, and to storage modules.
This wall had no designated function, so it was used for eclectic purposes, such as storing a laptop, an antibacterial experiment and resealable bags. And for 52 days during SQuARE, it was also the location where one crew member kept their toiletry kit.
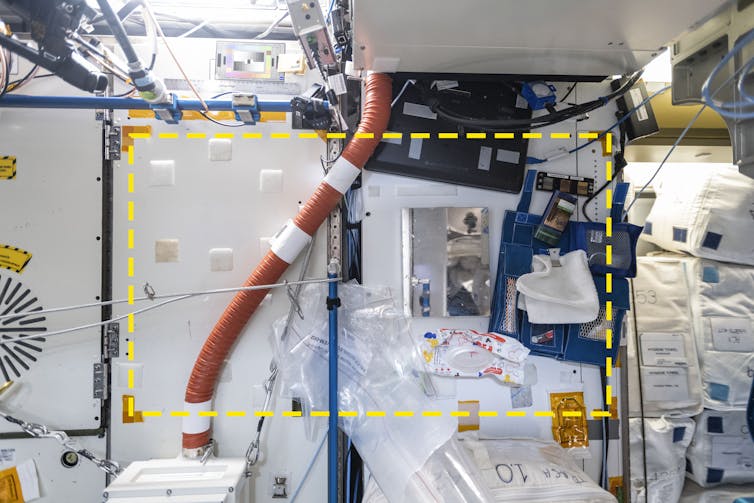
It makes a kind of sense to put one’s toiletries near the toilet and the exercise machines that each astronaut uses for hours every day. But this is a highly public space, where others are constantly passing by. The placement of the toiletry kit shows how inadequate the facilities are for hygiene and privacy.
What does this mean?
Our analysis of Squares 03 and 05 helped us understand how restraints such as velcro create a sort of transient gravity.
Restraints used to hold an object form a patch of active gravity, while those not in use represent potential gravity. The artefact analysis shows us how much potential gravity is available at each location.
The main focus of the space station is scientific work. To make this happen, astronauts have to deploy large numbers of objects. Square 03 shows how they turned a surface intended for maintenance into a halfway house for various items on their journeys around the station.
Our data suggests that designers of future space stations, such as the commercial ones currently planned for low Earth orbit, or the Gateway station being built for lunar orbit, might need to make storage a higher priority.
Square 05 shows how a public wall space was claimed for personal storage by an unknown crew member. We already know there is less-than-ideal provision for privacy, but the persistence of the toiletry bag at this location shows how crew adapt spaces to make up for this.
What makes our conclusions significant is that they are evidence-based. The analysis of the first two squares suggests the data from all six will offer further insights into humanity’s longest surviving space habitat.
Current plans are to bring the space station down from orbit in 2031, so this experiment may be the only chance we have to gather archaeological data.
The authors gratefully acknowledge the work of our collaborators Shawn Graham, Chantal Brousseau, and Salma Abdullah.
- Archaeology
- Spaceflight
- Life in space
- International Space Station
- Human Spaceflight

Senior Laboratory Technician

Manager, Centre Policy and Translation

Newsletter and Deputy Social Media Producer

College Director and Principal | Curtin College

Head of School: Engineering, Computer and Mathematical Sciences
Click through the PLOS taxonomy to find articles in your field.
For more information about PLOS Subject Areas, click here .
Loading metrics
Open Access
Peer-reviewed
Research Article
Archaeology in space: The Sampling Quadrangle Assemblages Research Experiment (SQuARE) on the International Space Station. Report 1: Squares 03 and 05
Roles Conceptualization, Data curation, Formal analysis, Funding acquisition, Investigation, Methodology, Project administration, Resources, Supervision, Visualization, Writing – original draft, Writing – review & editing
* E-mail: [email protected]
Affiliations Department of Art, Chapman University, Orange, CA, United States of America, Space Engineering Research Center, University of Southern California, Marina del Rey, CA, United States of America

Roles Data curation, Formal analysis, Investigation, Methodology, Resources, Software, Supervision, Validation, Visualization, Writing – original draft, Writing – review & editing
Affiliation Department of History, Carleton University, Ottawa, ON, United States of America
Roles Conceptualization, Data curation, Methodology, Project administration, Supervision, Writing – review & editing
Affiliation College of Humanities, Arts and Social Sciences, Flinders University, Adelaide, Australia
Roles Software, Writing – original draft
Roles Investigation, Writing – original draft
Affiliation Archaeology Research Center, University of Southern California, Los Angeles, CA, United States of America
- Justin St. P. Walsh,
- Shawn Graham,
- Alice C. Gorman,
- Chantal Brousseau,
- Salma Abdullah
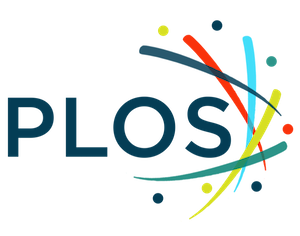
- Published: August 7, 2024
- https://doi.org/10.1371/journal.pone.0304229
- Reader Comments
Between January and March 2022, crew aboard the International Space Station (ISS) performed the first archaeological fieldwork in space, the Sampling Quadrangle Assemblages Research Experiment (SQuARE). The experiment aimed to: (1) develop a new understanding of how humans adapt to life in an environmental context for which we are not evolutionarily adapted, using evidence from the observation of material culture; (2) identify disjunctions between planned and actual usage of facilities on a space station; (3) develop and test techniques that enable archaeological research at a distance; and (4) demonstrate the relevance of social science methods and perspectives for improving life in space. In this article, we describe our methodology, which involves a creative re-imagining of a long-standing sampling practice for the characterization of a site, the shovel test pit. The ISS crew marked out six sample locations (“squares”) around the ISS and documented them through daily photography over a 60-day period. Here we present the results from two of the six squares: an equipment maintenance area, and an area near exercise equipment and the latrine. Using the photographs and an innovative webtool, we identified 5,438 instances of items, labeling them by type and function. We then performed chronological analyses to determine how the documented areas were actually used. Our results show differences between intended and actual use, with storage the most common function of the maintenance area, and personal hygiene activities most common in an undesignated area near locations for exercise and waste.
Citation: Walsh JSP, Graham S, Gorman AC, Brousseau C, Abdullah S (2024) Archaeology in space: The Sampling Quadrangle Assemblages Research Experiment (SQuARE) on the International Space Station. Report 1: Squares 03 and 05. PLoS ONE 19(8): e0304229. https://doi.org/10.1371/journal.pone.0304229
Editor: Peter F. Biehl, University of California Santa Cruz, UNITED STATES OF AMERICA
Received: March 9, 2024; Accepted: May 7, 2024; Published: August 7, 2024
Copyright: © 2024 Walsh et al. This is an open access article distributed under the terms of the Creative Commons Attribution License , which permits unrestricted use, distribution, and reproduction in any medium, provided the original author and source are credited.
Data Availability: All relevant data are within the paper and its Supporting Information files.
Funding: JW was the recipient of funding from Chapman University’s Office of Research and Sponsored Programs to support the activities of Axiom Space as implementation partner for the research presented in this article. There are no associated grant numbers for this financial support. Axiom Space served in the role of a contractor hired by Chapman University for the purpose of overseeing logistics relating to our research. In-kind support in the form of ISS crew time and access to the space station’s facilities, also awarded to JW from the ISS National Laboratory, resulted from an unsolicited proposal, and therefore there is no opportunity title or number associated with our work. No salary was received by any of the investigators as a result of the grant support. No additional external funding was received for this study.
Competing interests: The authors have declared that no competing interests exist.
Introduction
The International Space Station Archaeological Project (ISSAP) aims to fill a gap in social science investigation into the human experience of long-duration spaceflight [ 1 – 3 ]. As the largest, most intensively inhabited space station to date, with over 270 visitors from 23 countries during more than 23 years of continuous habitation, the International Space Station (ISS) is the ideal example of a new kind of spacefaring community—“a microsociety in a miniworld” [ 4 ]. While it is possible to interview crew members about their experiences, the value of an approach focused on material culture is that it allows identification of longer-term patterns of behaviors and associations that interlocutors are unable or even unwilling to articulate. In this respect, we are inspired by previous examples of contemporary archaeology such as the Tucson Garbage Project and the Undocumented Migration Project [ 5 – 7 ]. We also follow previous discussions of material culture in space contexts that highlight the social and cultural features of space technology [ 8 , 9 ].
Our primary goal is to identify how humans adapt to life in a new environment for which our species has not evolved, one characterized by isolation, confinement, and especially microgravity. Microgravity introduces opportunities, such as the ability to move and work in 360 degrees, and to carry out experiments impossible in full Earth gravity, but also limitations, as unrestrained objects float away. The most routine activities carried out on Earth become the focus of intense planning and technological intervention in microgravity. By extension, our project also seeks to develop archaeological techniques that permit the study of other habitats in remote, extreme, or dangerous environments [ 10 , 11 ]. Since it is too costly and difficult to visit our archaeological site in person, we have to creatively re-imagine traditional archaeological methods to answer key questions. To date, our team has studied crew-created visual displays [ 12 , 13 ], meanings and processes associated with items returned to Earth [ 14 ], distribution of different population groups around the various modules [ 15 ], and the development of machine learning (ML) computational techniques to extract data about people and places, all from historic photographs of life on the ISS [ 16 ].
From January to March 2022, we developed a new dataset through the first archaeological work conducted off-Earth. We documented material culture in six locations around the ISS habitat, using daily photography taken by the crew which we then annotated and studied as evidence for changes in archaeological assemblages of material culture over time. This was the first time such data had been captured in a way that allowed statistical analysis. Here, we present the data and results from Squares 03 and 05, the first two sample locations to be completed.
Materials and methods
Square concept and planning.
Gorman proposed the concept behind the investigation, deriving it from one of the most traditional terrestrial archaeological techniques, the shovel test pit. This method is used to understand the overall characteristics of a site quickly through sampling. A site is mapped with a grid of one-meter squares. Some of the squares are selected for initial excavation to understand the likely spatial and chronological distribution of features across the entire site. In effect, the technique is a way to sample a known percentage of the entire site systematically. In the ISS application of this method, we documented a notional stratigraphy through daily photography, rather than excavation.
Historic photography is a key dataset for the International Space Station Archaeological Project. Tens of thousands of images have been made available to us, either through publication [ 17 ], or through an arrangement with the ISS Research Integration Office, which supplied previously unpublished images from the first eight years of the station’s habitation. These photographs are informative about the relationships between people, places, and objects over time in the ISS. However, they were taken randomly (from an archaeological perspective) and released only according to NASA’s priorities and rules. Most significantly, they were not made with the purpose of answering archaeological questions. By contrast, the photographs taken during the present investigation were systematic, representative of a defined proportion of the habitat’s area, and targeted towards capturing archaeology’s primary evidence: material culture. We were interested in how objects move around individual spaces and the station, what these movements revealed about crew adherence to terrestrial planning, and the creative use of material culture to make the laboratory-like interior of the ISS more habitable.
Access to the field site was gained through approval of a proposal submitted to the Center for the Advancement of Science in Space (also known as the ISS National Laboratory [ISS NL]). Upon acceptance, Axiom Space was assigned as the Implementation Partner for carriage of the experiment according to standard procedure. No other permits were required for this work.
Experiment design
Since our work envisioned one-meter sample squares, and recognizing the use of acronyms as a persistent element of spacefaring culture, we named our payload the Sampling Quadrangle Assemblages Research Experiment (SQuARE). Permission from the ISS NL to conduct SQuARE was contingent on using equipment that was already on board the space station. SQuARE required only five items: a camera, a wide-angle lens, adhesive tape (for marking the boundaries of the sample locations), a ruler (for scale), and a color calibration card (for post-processing of the images). All of these were already present on the ISS.
Walsh performed tests on the walls of a terrestrial art gallery to assess the feasibility of creating perfect one-meter squares in microgravity. He worked on a vertical surface, using the Pythagorean theorem to determine where the corners should be located. The only additional items used for these tests were two metric measuring tapes and a pencil for marking the wall (these were also already on the ISS). While it was possible to make a square this way, it also became clear that at least two people were needed to manage holding the tape measures in position while marking the points for the corners. This was not possible in the ISS context.
Walsh and Gorman identified seven locations for the placement of squares. Five of these were in the US Orbital Segment (USOS, consisting of American, European, and Japanese modules) and two in the Russian Orbital Segment. Unfortunately, tense relations between the US and Russian governments meant we could only document areas in the USOS. The five locations were (with their SQuARE designations):
- 01—an experimental rack on the forward wall, starboard end, of the Japanese Experiment Module
- 02—an experimental rack on the forward wall, port end, of the European laboratory module Columbus
- 03—the starboard Maintenance Work Area (workstation) in the US Node 2 module
- 04—the wall area “above” (according to typical crew body orientation) the galley table in the US Node 1 module
- 05—the aft wall, center location, of the US Node 3 module
Our square selection encompassed different modules and activities, including work and leisure. We also asked the crew to select a sixth sample location based on their understanding of the experiment and what they thought would be interesting to document. They chose a workstation on the port wall of the US laboratory module, at the aft end, which they described in a debriefing following their return to Earth in June 2022 as “our central command post, like our shared office situation in the lab.” Results from the four squares not included here will appear in future publications.
Walsh worked with NASA staff to determine payload procedures, including precise locations for the placement of the tape that would mark the square boundaries. The squares could not obstruct other facilities or experiments, so (unlike in terrestrial excavations, where string is typically used to demarcate trench boundaries) only the corners of each square were marked, not the entire perimeter. We used Kapton tape due to its bright yellow-orange color, which aided visibility for the crew taking photographs and for us when cropping the images. In practice, due to space constraints, the procedures that could actually be performed by crew in the ISS context, and the need to avoid interfering with other ongoing experiments, none of the locations actually measured one square meter or had precise 90° corners like a trench on Earth.
On January 14, 2022, NASA astronaut Kayla Barron set up the sample locations, marking the beginning of archaeological work in space ( S1 Movie ). For 30 days, starting on January 21, a crew member took photos of the sample locations at approximately the same time each day; the process was repeated at a random time each day for a second 30-day period to eliminate biases. Photography ended on March 21, 2022. The crew were instructed not to move any items prior to taking the photographs. Walsh led image management, including color and barrel distortion correction, fixing the alignment of each image, and cropping them to the boundaries of the taped corners.
Data processing—Item tagging, statistics, visualizations
We refer to each day’s photo as a “context” by analogy with chronologically-linked assemblages of artifacts and installations at terrestrial archaeological sites ( S1 and S2 Datasets). As previously noted, each context represented a moment roughly 24 hours distant from the previous one, showing evidence of changes in that time. ISS mission planners attempted to schedule the activity at the same time in the first month, but there were inevitable changes due to contingencies. Remarkably, the average time between contexts in Phase 1 was an almost-perfect 24h 0m 13s. Most of the Phase 1 photos were taken between 1200 and 1300 GMT (the time zone in which life on the ISS is organized). In Phase 2, the times were much more variable, but the average time between contexts during this period was still 23h 31m 45s. The earliest Phase 2 photo was taken at 0815 GMT, and the latest at 2101. We did not identify any meaningful differences between results from the two phases.
Since the “test pits” were formed of images rather than soil matrices, we needed a tool to capture information about the identity, nature, and location of every object. An open-source image annotator platform [ 18 ] mostly suited our needs. Brousseau rebuilt the platform to work within the constraints of our access to the imagery (turning it into a desktop tool with secure access to our private server), to permit a greater range of metadata to be added to each item or be imported, to autosave, and to export the resulting annotations. The tool also had to respect privacy and security limitations required by NASA.
The platform Brousseau developed and iterated was rechristened “Rocket-Anno” ( S1 File ). For each context photograph, the user draws an outline around every object, creating a polygon; each polygon is assigned a unique ID and the user provides the relevant descriptive information, using a controlled vocabulary developed for ISS material culture by Walsh and Gorman. Walsh and Abdullah used Rocket-Anno to tag the items in each context for Squares 03 and 05. Once all the objects were outlined for every context’s photograph, the tool exported a JSON file with all of the metadata for both the images themselves and all of the annotations, including the coordinate points for every polygon ( S3 Dataset ). We then developed Python code using Jupyter “notebooks” (an interactive development environment) that ingests the JSON file and generates dataframes for various facets of the data. Graham created a “core” notebook that exports summary statistics, calculates Brainerd-Robinson coefficients of similarity, and visualizes the changing use of the square over time by indicating use-areas based on artifact types and subtypes ( S2 File ). Walsh and Abdullah also wrote detailed square notes with context-by-context discussions and interpretations of features and patterns.
We asked NASA for access to the ISS Crew Planner, a computer system that shows each astronaut’s tasks in five-minute increments, to aid with our interpretation of contexts, but were denied. As a proxy, we use another, less detailed source: the ISS Daily Summary Reports (DSRs), published on a semi-regular basis by NASA on its website [ 19 ]. Any activities mentioned in the DSRs often must be connected with a context by inference. Therefore, our conclusions are likely less precise than if we had seen the Crew Planner, but they also more clearly represent the result of simply observing and interpreting the material culture record.
The crew during our sample period formed ISS Expedition 66 (October 2021-March 2022). They were responsible for the movement of objects in the sample squares as they carried out their daily tasks. The group consisted of two Russians affiliated with Roscosmos (the Russian space agency, 26%), one German belonging to the European Space Agency (ESA, 14%), and four Americans employed by NASA (57%). There were six men (86%) and one woman (14%), approximately equivalent to the historic proportions in the ISS population (84% and 16%, respectively). The Russian crew had their sleeping quarters at the aft end of the station, in the Zvezda module. The ESA astronaut slept in the European Columbus laboratory module. The four NASA crew slept in the US Node 2 module (see below). These arrangements emphasize the national character of discrete spaces around the ISS, also evident in our previous study of population distributions [ 15 ]. Both of the sample areas in this study were located in US modules.
Square 03 was placed in the starboard Maintenance Work Area (MWA, Fig 1 ), one of a pair of workstations located opposite one another in the center of the Node 2 module, with four crew berths towards the aft and a series of five ports for the docking of visiting crew/cargo vehicles and two modules on the forward end ( Fig 2 ). Node 2 (sometimes called “Harmony”) is a connector that links the US, Japanese, and European lab modules. According to prevailing design standards when the workstation was developed, an MWA “shall serve as the primary location for servicing and repair of maximum sized replacement unit/system components” [ 20 ]. Historic images published by NASA showing its use suggested that its primary function was maintenance of equipment and also scientific work that did not require a specific facility such as a centrifuge or furnace.
- PPT PowerPoint slide
- PNG larger image
- TIFF original image
An open crew berth is visible at right. The yellow dotted line indicates the boundaries of the sample area. Credit: NASA/ISSAP.
https://doi.org/10.1371/journal.pone.0304229.g001
Credit: Tor Finseth, by permission, modified by Justin Walsh.
https://doi.org/10.1371/journal.pone.0304229.g002
Square 03 measured 90.3 cm (top) x 87.8 (left) x 89.4 (bottom) x 87.6 (right), for an area of approximately 0.79 m 2 . Its primary feature was a blue metal panel with 40 square loop-type Velcro patches arranged in four rows of ten. During daily photography, many items were attached to the Velcro patches (or held by a clip or in a resealable bag which had its own hook-type Velcro). Above and below the blue panel were additional Velcro patches placed directly on the white plastic wall surface. These patches were white, in different sizes and shapes and irregularly arranged, indicating that they had been placed on the wall in response to different needs. Some were dirty, indicating long use. The patches below the blue panel were rarely used during the sample period, but the patches above were used frequently to hold packages of wet wipes, as well as resealable bags with electrostatic dispersion kits and other items. Outside the sample area, the primary features were a crew berth to the right, and a blue metal table attached to the wall below. This table, the primary component of the MWA, “provides a rigid surface on which to perform maintenance tasks,” according to NASA [ 21 ]. It is modular and can be oriented in several configurations, from flat against the wall to horizontal ( i . e ., perpendicular to the wall). A laptop to the left of the square occasionally showed information about work happening in the area.
In the 60 context photos of Square 03, we recorded 3,608 instances of items, an average of 60.1 (median = 60.5) per context. The lowest count was 24 in context 2 (where most of the wall was hidden from view behind an opaque storage bag), and the highest was 75 in both contexts 20 and 21. For comparison between squares, we can also calculate the item densities per m 2 . The average count was 76.1/m 2 (minimum = 30, maximum = 95). The count per context ( Fig 3(A)) began much lower than average in the first three contexts because of a portable glovebag and a stowage bag that obscured much of the sample square. It rose to an above-average level which was sustained (with the exception of contexts 11 and 12, which involved the appearance of another portable glovebag) until about context 43, when the count dipped again and the area seemed to show less use. Contexts 42–59 showed below-average numbers, as much as 20% lower than previously.
(a) Count of artifacts in Square 03 over time. (b) Proportions of artifacts by function in Square 03. Credit: Rao Hamza Ali.
https://doi.org/10.1371/journal.pone.0304229.g003
74 types of items appeared at least once here, belonging to six categories: equipment (41%), office supplies (31%), electronic (17%), stowage (9%), media (1%), and food (<1%). To better understand the significance of various items in the archaeological record, we assigned them to functional categories ( Table 1 , Fig 3(B)) . 35% of artifacts were restraints, or items used for holding other things in place; 12% for tools; 9% for containers; 9% for writing items; 6% for audiovisual items; 6% for experimental items; 4% for lights; 4% for safety items; 4% for body maintenance; 4% for power items; 3% for computing items; 1% for labels; and less than 1% drinks. We could not identify a function for two percent of the items.
https://doi.org/10.1371/journal.pone.0304229.t001
One of the project goals is understanding cultural adaptations to the microgravity environment. We placed special attention on “gravity surrogates,” pieces of (often simple) technology that are used in space to replicate the terrestrial experience of things staying where they are placed. Gravity surrogates include restraints and containers. It is quite noticeable that gravity surrogates comprise close to half of all items (44%) in Square 03, while the tools category, which might have been expected to be most prominent in an area designated for maintenance, is less than one-third as large (12%). Adding other groups associated with work, such as “experiment” and “light,” only brings the total to 22%.
Square 05 (Figs 2 and 4 ) was placed in a central location on the aft wall of the multipurpose Node 3 (“Tranquility”) module. This module does not include any specific science facilities. Instead, there are two large pieces of exercise equipment, the TVIS (Treadmill with Vibration Isolation Stabilization System, on the forward wall at the starboard end), and the ARED (Advanced Resistive Exercise Device, on the overhead wall at the port end). Use of the machines forms a significant part of crew activities, as they are required to exercise for two hours each day to counteract loss of muscle mass and bone density, and enable readjustment to terrestrial gravity on their return. The Waste and Hygiene Compartment (WHC), which includes the USOS latrine, is also here, on the forward wall in the center of the module, opposite Square 05. Finally, three modules are docked at Node 3’s port end. Most notable is the Cupola, a kind of miniature module on the nadir side with a panoramic window looking at Earth. This is the most popular leisure space for the crew, who often describe the hours they spend there. The Permanent Multipurpose Module (PMM) is docked on the forward side, storing equipment, food, and trash. In previous expeditions, some crew described installing a curtain in the PMM to create a private space for changing clothes and performing body maintenance activities such as cleaning oneself [ 22 , 23 ], but it was unclear whether that continued to be its function during the expedition we observed. One crew member during our sample period posted a video on Instagram showing the PMM interior and their efforts to re-stow equipment in a bag [ 24 ]. The last space attached to Node 3 is an experimental inflatable module docked on the aft side, called the Bigelow Expandable Activity Module (BEAM), which is used for storage of equipment.
The yellow dotted line indicates the boundaries of the sample area. The ARED machine is at the far upper right, on the overhead wall. The TVIS treadmill is outside this image to the left, on the forward wall. The WHC is directly behind the photographer. Credit: NASA/ISSAP.
https://doi.org/10.1371/journal.pone.0304229.g004
Square 05 was on a mostly featureless wall, with a vertical handrail in the middle. Handrails are metal bars located throughout the ISS that are used by the crew to hold themselves in place or provide a point from which to propel oneself to another location. NASA’s most recent design standards acknowledge that “[t]hey also serve as convenient locations for temporary mounting, affixing, or restraint of loose equipment and as attachment points for equipment” [ 25 ]. The handrail in Square 05 was used as an impromptu object restraint when a resealable bag filled with other bags was squeezed between the handrail and the wall.
The Brine Processing Assembly (BPA), a white plastic box which separates water from other components of urine for treatment and re-introduction to the station’s drinkable water supply [ 26 ], was fixed to the wall outside the square boundaries at lower left. A bungee cord was attached to both sides of the box; the one on the right was connected at its other end to the handrail attachment bracket. Numerous items were attached to or wedged into this bungee cord during the survey, bringing “gravity” into being. A red plastic duct ran through the square from top center into the BPA. This duct led from the latrine via the overhead wall. About halfway through the survey period, in context 32, the duct was wrapped in Kapton tape. According to the DSR for that day, “the crew used duct tape [ sic ] to make a seal around the BPA exhaust to prevent odor permeation in the cabin” [ 27 ], revealing an aspect of the crew’s experience of this area that is captured only indirectly in the context photograph. Permanently attached to the wall were approximately 20 loop-type Velcro patches in many shapes and sizes, placed in a seemingly random pattern that likely indicates that they were put there at different times and for different reasons.
Other common items in Square 05 were a mirror, a laptop computer, and an experimental item belonging to the German space agency DLR called the Touch Array Assembly [ 28 ]. The laptop moved just three times, and only by a few centimeters each time, during the sample period. The Touch Array was a black frame enclosing three metal surfaces which were being tested for their bacterial resistance; members of the crew touched the surfaces at various moments during the sample period. Finally, and most prominent due to its size, frequency of appearance, and use (judged by its movement between context photos) was an unidentified crew member’s toiletry kit.
By contrast with Square 03, 05 was the most irregular sample location, roughly twice as wide as it was tall. Its dimensions were 111 cm (top) x 61.9 (left) x 111.4 (bottom) x 64.6 (right), for an area of approximately 0.7 m 2 , about 89% of Square 03. We identified 1,830 instances of items in the 60 contexts, an average of 30.5 (median = 32) per context. The minimum was 18 items in context 5, and the maximum was 39 in contexts 24, 51, and 52. The average item density was 43.6/m 2 (minimum = 26, maximum = 56), 57% of Square 03.
The number of items trended upward throughout the sample period ( Fig 5(A)) . The largest spike occurred in context 6 with the appearance of the toiletry kit, which stored (and revealed) a number of related items. The kit can also be linked to one of the largest dips in item count, seen from contexts 52 to 53, when it was closed (but remained in the square). Other major changes can often be attributed to the addition and removal of bungee cords, which had other items such as carabiners and brackets attached. For example, the dip seen in context 25 correlates with the removal of a bungee cord with four carabiners.
(a) Count of artifacts and average count in Square 05 over time. (b) Proportions of artifacts by function in Square 05. Credit: Rao Hamza Ali.
https://doi.org/10.1371/journal.pone.0304229.g005
41 different item types were found in Square 05, about 55% as many as in Square 03. These belonged to five different categories: equipment (63%), electronic (17%), stowage (10%), office supplies (5%), and food (2%). The distribution of function proportions was quite different in this sample location ( Table 2 and Fig 5(B)) . Even though restraints were still most prominent, making up 32% of all items, body maintenance was almost as high (30%), indicating how strongly this area was associated with the activity of cleaning and caring for oneself. Computing (8%, represented by the laptop, which seems not to have been used), power (8%, from various cables), container (7%, resealable bags and Cargo Transfer Bags), and hygiene (6%, primarily the BPA duct) were the next most common items. Experiment was the function of 4% of the items, mostly the Touch Array, which appeared in every context, followed by drink (2%) and life support (1%). Safety, audiovisual, food, and light each made up less than 1% of the functional categories.
https://doi.org/10.1371/journal.pone.0304229.t002
Tracking changes over time is critical to understanding the activity happening in each area. We now explore how the assemblages change by calculating the Brainerd-Robinson Coefficient of Similarity [ 29 , 30 ] as operationalized by Peeples [ 31 , 32 ]. This metric is used in archaeology for comparing all pairs of the contexts by the proportions of categorical artifact data, here functional type. Applying the coefficient to the SQuARE contexts enables identification of time periods for distinct activities using artifact function and frequency alone, independent of documentary or oral evidence.
Multiple phases of activities took place in the square. Moments of connected activity are visible as red clusters in contexts 0–2, 11–12, 28–32, and 41 ( Fig 6(A)) . Combining this visualization with close observation of the photos themselves, we argue that there are actually eight distinct chronological periods.
- Contexts 0–2: Period 1 (S1 Fig in S3 File ) is a three-day period of work involving a portable glovebag (contexts 0–1) and a large blue stowage bag (context 2). It is difficult to describe trends in functional types because the glovebag and stowage bag obstruct the view of many objects. Items which appear at the top of the sample area, such as audiovisual and body maintenance items, are overemphasized in the data as a result. It appears that some kind of science is happening here, perhaps medical sample collection due to the presence of several small resealable bags visible in the glovebag. The work appears particularly intense in context 1, with the positioning of the video camera and light to point into the glovebag. These items indicate observation and oversight of crew activities by ground control. A white cargo transfer bag for storage and the stowage bag for holding packing materials in the context 2 photo likely relate to the packing of a Cargo Dragon vehicle that was docked to Node 2. The Dragon departed from the ISS for Earth, full of scientific samples, equipment, and crew personal items, a little more than three hours after the context 2 photo was taken [ 33 ].
- Contexts 3–10: Period 2 (S2 Fig in S3 File ) was a “stable” eight-day period in the sample, when little activity is apparent, few objects were moved or transferred in or out the square, and the primary function of the area seems to be storage rather than work. In context 6, a large Post-It notepad appeared in the center of the metal panel with a phone number written on it. This number belonged to another astronaut, presumably indicating that someone on the ISS had been told to call that colleague on the ground (for reasons of privacy, and in accordance with NASA rules for disseminating imagery, we have blurred the number in the relevant images). In context 8, the same notepad sheet had new writing appear on it, this time reading “COL A1 L1,” the location of an experimental rack in the European lab module.
- Contexts 11–12: Period 3 (S3 Fig in S3 File ) involves a second appearance of a portable glovebag (a different one from that used in contexts 0–1, according to its serial number), this time for a known activity, a concrete hardening experiment belonging to the European Space Agency [ 34 , 35 ]. This two-day phase indicates how the MWA space can be shared with non-US agencies when required. It also demonstrates the utility of this flexible area for work beyond biology/medicine, such as material science. Oversight of the crew’s activities by ground staff is evident from the positioning of the video camera and LED light pointing into the glovebag.
- Contexts 13–27: Period 4 (S4 Fig in S3 File ) is another stable fifteen-day period, similar to Period 2. Many items continued to be stored on the aluminum panel. The LED light’s presence is a trace of the activity in Period 3 that persists throughout this phase. Only in context 25 can a movement of the lamp potentially be connected to an activity relating to one of the stored items on the wall: at least one nitrile glove was removed from a resealable bag behind the lamp. In general, the primary identifiable activity during Period 4 is storage.
- Contexts 28–32: Period 5 (S5 Fig in S3 File ), by contrast, represents a short period of five days of relatively high and diverse activity. In context 28, a Microsoft Hololens augmented reality headset appeared. According to the DSR for the previous day, a training activity called Sidekick was carried out using the headset [ 36 ]. The following day, a Saturday, showed no change in the quantity or type of objects, but many were moved around and grouped by function—adhesive tape rolls were placed together, tools were moved from Velcro patches into pouches or straightened, and writing implements were placed in a vertical orientation when previously they were tilted. Context 29 represents a cleaning and re-organization of the sample area, which is a common activity for the crew on Saturdays [ 37 ]. Finally, in context 32, an optical coherence tomography scanner—a large piece of equipment for medical research involving crew members’ eyes—appeared [ 38 ]. This device was used previously during the sample period, but on the same day as the ESA concrete experiment, so that earlier work seems to have happened elsewhere [ 39 ].
- Contexts 33–40: Period 6 (S6 Fig in S3 File ) is the third stable period, in which almost no changes are visible over eight days. The only sign of activity is a digital timer which was started six hours before the context 39 image was made and continued to run at least through context 42.
- Context 41: Period 7 (S7 Fig in S3 File ) is a single context in which medical sample collection may have occurred. Resealable bags (some holding others) appeared in the center of the image and at lower right. One of the bags at lower right had a printed label reading “Reservoir Containers.” We were not able to discern which type of reservoir containers the label refers to, although the DSR for the day mentions “[Human Research Facility] Generic Saliva Collection,” without stating the location for this work [ 40 ]. Evidence from photos of other squares shows that labeled bags could be re-used for other purposes, so our interpretation of medical activity for this context is not conclusive.
- Contexts 42–60: Period 8 (S8 Fig in S3 File ) is the last and longest period of stability and low activity—eighteen days in which no specific activity other than the storage of items can be detected. The most notable change is the appearance for the first time of a foil water pouch in the central part of the blue panel.
Visualization of Brainerd-Robinson similarity, compared context-by-context by item function, for (a) Square 03 and (b) Square 05. The more alike a pair of contexts is, the higher the coefficient value, with a context compared against itself where a value of 200 equals perfect similarity. The resulting matrix of coefficients is visualized on a scale from blue to red where blue is lowest and red is highest similarity. The dark red diagonal line indicates complete similarity, where each context is compared to itself. Dark blue represents a complete difference. Credit: Shawn Graham.
https://doi.org/10.1371/journal.pone.0304229.g006
In the standards used at the time of installation, “stowage space” was the sixth design requirement listed for the MWA after accessibility; equipment size capability; scratch-resistant surfaces; capabilities for electrical, mechanical, vacuum, and fluid support during maintenance; and the accommodation of diagnostic equipment [ 20 ]. Only capabilities for fabrication were listed lower than stowage. Yet 50 of the 60 contexts (83%) fell within stable periods where little or no activity is identifiable in Square 03. According to the sample results, therefore, this area seems to exist not for “maintenance,” but primarily for the storage and arrangement of items. The most recent update of the design standards does not mention the MWA, but states, “Stowage location of tool kits should be optimized for accessibility to workstations and/or maintenance workbenches” [ 25 ]. Our observation confirms the importance of this suggestion.
The MWA was also a flexible location for certain science work, like the concrete study or crew health monitoring. Actual maintenance of equipment was hardly in evidence in the sample (possibly contexts 25, 39, and 44), and may not even have happened at all in this location. Some training did happen here, such as review of procedures for the Electromagnetic Levitator camera (instructions for changing settings on a high-speed camera appeared on the laptop screen; the day’s DSR shows that this camera is part of the Electromagnetic Levitator facility, located in the Columbus module [ 41 ]. The training required the use of the Hololens system (context 28 DSR, cited above).
Although many item types were represented in Square 03, it became clear during data capture how many things were basically static, unmoving and therefore unused, especially certain tools, writing implements, and body maintenance items. The MWA was seen as an appropriate place to store these items. It may be the case that their presence here also indicates that their function was seen as an appropriate one for this space, but the function(s) may not be carried out—or perhaps not in this location. Actualization of object function was only visible to us when the state of the item changed—it appeared, it moved, it changed orientation, it disappeared, or, in the case of artifacts that were grouped in collections rather than found as singletons, its shape changed or it became visibly smaller/lesser. We therefore have the opportunity to explore not only actuality of object use, but also potentiality of use or function, and the meaning of that quality for archaeological interpretation [ 42 , 43 ]. This possibility is particularly intriguing in light of the archaeological turn towards recognizing the agency of objects to impact human activity [ 44 , 45 ]. We will explore these implications in a future publication.
We performed the same chronological analysis for Square 05. Fig 6(B) represents the analysis for both item types and for item functions. We identified three major phases of activity, corresponding to contexts 0–5, 6–52, and 53–59 (S9-S11 Figs in S3 File ). The primary characteristics of these phases relate to an early period of unclear associations (0–5) marked by the presence of rolls of adhesive tape and a few body maintenance items (toothpaste and toothbrush, wet wipes); the appearance of a toiletry kit on the right side of the sample area, fully open with clear views of many of the items contained within (6–52); and finally, the closure of the toiletry kit so that its contents can no longer be seen (53–59). We interpret the phases as follows:
- Contexts 0–5: In Period 1 (six days, S9 Fig in S3 File ), while items such as a mirror, dental floss picks, wet wipes, and a toothbrush held in the end of a toothpaste tube were visible, the presence of various other kinds of items confounds easy interpretation. Two rolls of duct tape were stored on the handrail in the center of the sample area, and the Touch Array and laptop appeared in the center. Little movement can be identified, apart from a blue nitrile glove that appeared in context 1 and moved left across the area until it was wedged into the bungee cord for contexts 3 and 4. The tape rolls were removed prior to context 5. A collection of resealable bags was wedged behind the handrail in context 3, remaining there until context 9. Overall, this appears to be a period characterized by eclectic associations, showing an area without a clear designated function.
- Contexts 6–52: Period 2 (S10 Fig in S3 File ) is clearly the most significant one for this location due to its duration (47 days). It was dominated by the number of body maintenance items located in and around the toiletry kit, especially a white hand towel (on which a brown stain was visible from context 11, allowing us to confirm that the same towel was present until context 46). A second towel appeared alongside the toiletry kit in context 47, and the first one was fixed at the same time to the handrail, where it remained through the end of the sample period. A third towel appeared in context 52, attached to the handrail together with the first one by a bungee cord, continuing to the end of the sample period. Individual body maintenance items moved frequently from one context to the next, showing the importance of this type of activity for this part of Node 3. For reasons that are unclear, the mirror shifted orientation from vertical to diagonal in context 22, and then was put back in a vertical orientation in context 31 (a Monday, a day which is not traditionally associated with cleaning and organization). Collections of resealable bags appeared at various times, including a large one labeled “KYNAR BAG OF ZIPLOCKS” in green marker at the upper left part of the sample area beginning of context 12 (Kynar is a non-flammable plastic material that NASA prefers for resealable bags to the generic commercial off-the-shelf variety because it is non-flammable; however, its resistance to heat makes it less desirable for creating custom sizes, so bags made from traditional but flammable low-density polyethylene still dominate on the ISS [ 14 ]). The Kynar bag contained varying numbers of bags within it over time; occasionally, it appeared to be empty. The Touch Array changed orientation on seven of 47 days in period 2, or 15% of the time (12% of all days in the survey), showing activity associated with scientific research in this area. In context 49, a life-support item, the Airborne Particulate Monitor (APM) was installed [ 46 ]. This device, which measures “real-time particulate data” to assess hazards to crew health [ 47 ], persisted through the end of the sample period.
- Contexts 53–59: Period 3 (S11 Fig in S3 File ) appears as a seven-day phase marked by low activity. Visually, the most notable feature is the closure of the toiletry kit, which led to much lower counts of body maintenance items. Hardly any of the items on the wall moved at all during this period.
While body maintenance in the form of cleaning and caring for oneself could be an expected function for an area with exercise and excretion facilities, it is worth noting that the ISS provides, at most, minimal accommodation for this activity. A description of the WHC stated, “To provide privacy…an enclosure was added to the front of the rack. This enclosure, referred to as the Cabin, is approximately the size of a typical bathroom stall and provides room for system consumables and hygiene item stowage. Space is available to also support limited hygiene functions such as hand and body washing” [ 48 ]. A diagram of the WHC in the same publication shows the Cabin without a scale but suggests that it measures roughly 2 m (h) x .75 (w) x .75 (d), a volume of approximately 1.125 m 3 . NASA’s current design standards state that the body volume of a 95th percentile male astronaut is 0.99 m 3 [ 20 ], meaning that a person of that size would take up 88% of the space of the Cabin, leaving little room for performing cleaning functions—especially if the Cabin is used as apparently intended, to also hold “system consumables and hygiene item[s]” that would further diminish the usable volume. This situation explains why crews try to adapt other spaces, such as storage areas like the PMM, for these activities instead. According to the crew debriefing statement, only one of them used the WHC for body maintenance purposes; it is not clear whether the toiletry kit belonged to that individual. But the appearance of the toiletry kit in Square 05—outside of the WHC, in a public space where others frequently pass by—may have been a response to the limitations of the WHC Cabin. It suggests a need for designers to re-evaluate affordances for body maintenance practices and storage for related items.
Although Square 03 and 05 were different sizes and shapes, comparing the density of items by function shows evidence of their usage ( Table 3 ). The typical context in Square 03 had twice as many restraints and containers, but less than one-quarter as many body maintenance items as Square 05. 03 also had many tools, lights, audiovisual equipment, and writing implements, while there were none of any of these types in 05. 05 had life support and hygiene items which were missing from 03. It appears that flexibility and multifunctionality were key elements for 03, while in 05 there was emphasis on one primary function (albeit an improvised one, designated by the crew rather than architects or ground control), cleaning and caring for one’s body, with a secondary function of housing static equipment for crew hygiene and life support.
https://doi.org/10.1371/journal.pone.0304229.t003
As this is the first time such an analysis has been performed, it is not yet possible to say how typical or unusual these squares are regarding the types of activities taking place; but they provide a baseline for eventual comparison with the other four squares and future work on ISS or other space habitats.
Some general characteristics are revealed by archaeological analysis of a space station’s material culture. First, even in a small, enclosed site, occupied by only a few people over a relatively short sample period, we can observe divergent patterns for different locations and activity phases. Second, while distinct functions are apparent for these two squares, they are not the functions that we expected prior to this research. As a result, our work fulfills the promise of the archaeological approach to understanding life in a space station by revealing new, previously unrecognized phenomena relating to life and work on the ISS. There is now systematically recorded archaeological data for a space habitat.
Squares 03 and 05 served quite different purposes. The reasons for this fact are their respective affordances and their locations relative to activity areas designated for science and exercise. Their national associations, especially the manifestation of the control wielded by NASA over its modules, also played a role in the use of certain materials, the placement of facilities, and the organization of work. How each area was used was also the result of an interplay between the original plans developed by mission planners and habitat designers (or the lack of such plans), the utility of the equipment and architecture in each location, and the contingent needs of the crew as they lived in the station. This interplay became visible in the station’s material culture, as certain areas were associated with particular behaviors, over time and through tradition—over the long duration across many crews (Node 2, location of Square 03, docked with the ISS in 2007, and Node 3, location of Square 05, docked in 2010), and during the specific period of this survey, from January to March 2022. During the crew debriefing, one astronaut said, “We were a pretty organized crew who was also pretty much on the same page about how to do things…. As time went on…we organized the lab and kind of got on the same page about where we put things and how we’re going to do things.” This statement shows how functional associations can become linked to different areas of the ISS through usage and mutual agreement. At the same time, the station is not frozen in time. Different people have divergent ideas about how and where to do things. It seems from the appearance of just one Russian item—a packet of generic wipes ( salfetky sukhiye ) stored in the toiletry kit throughout the sample period—that the people who used these spaces and carried out their functions did not typically include the ISS’s Russian crew. Enabling greater flexibility to define how spaces can be used could have a significant impact on improving crew autonomy over their lives, such as how and where to work. It could also lead to opening of all spaces within a habitat to the entire crew, which seems likely to improve general well-being.
An apparent disjunction between planned and actual usage appeared in Square 03. It is intended for maintenance as well as other kinds of work. But much of the time, there was nobody working here—a fact that is not captured by historic photos of the area, precisely because nothing is happening. The space has instead become the equivalent of a pegboard mounted on a wall in a home garage or shed, convenient for storage for all kinds of items—not necessarily items being used there—because it has an enormous number of attachment points. Storage has become its primary function. Designers of future workstations in space should consider that they might need to optimize for functions other than work, because most of the time, there might not be any work happening there. They could optimize for quick storage, considering whether to impose a system of organization, or allow users to organize as they want.
We expected from previous (though unsystematic) observation of historic photos and other research, that resealable plastic bags (combined with Velcro patches on the bags and walls) would be the primary means for creating gravity surrogates to control items in microgravity. They only comprise 7% of all items in Square 03 (256 instances). There are more than twice as many clips (572—more than 9 per context) in the sample. There were 193 instances of adhesive tape rolls, and more than 100 cable ties, but these were latent (not holding anything), representing potentiality of restraint rather than actualization. The squares showed different approaches to managing “gravity.” While Square 03 had a pre-existing structured array of Velcro patches, Square 05 showed a more expedient strategy with Velcro added in response to particular activities. Different needs require different affordances; creating “gravity” is a more nuanced endeavor than it initially appears. More work remains to be done to optimize gravity surrogates for future space habitats, because this is evidently one of the most critical adaptations that crews have to make in microgravity (44% of all items in Square 03, 39% in 05).
Square 05 is an empty space, seemingly just one side of a passageway for people going to use the lifting machine or the latrine, to look out of the Cupola, or get something out of deep storage in one of the ISS’s closets. In our survey, this square was a storage place for toiletries, resealable bags, and a computer that never (or almost never) gets used. It was associated with computing and hygiene simply by virtue of its location, rather than due to any particular facilities it possessed. It has no affordances for storage. There are no cabinets or drawers, as would be appropriate for organizing and holding crew personal items. A crew member decided that this was an appropriate place to leave their toiletry kit for almost two months. Whether this choice was appreciated or resented by fellow crew members cannot be discerned based on our evidence, but it seems to have been tolerated, given its long duration. The location of the other four USOS crew members’ toiletry kits during the sample period is unknown. A question raised by our observations is: how might a function be more clearly defined by designers for this area, perhaps by providing lockers for individual crew members to store their toiletries and towels? This would have a benefit not only for reducing clutter, but also for reducing exposure of toiletry kits and the items stored in them to flying sweat from the exercise equipment or other waste particles from the latrine. A larger compartment providing privacy for body maintenance and a greater range of motion would also be desirable.
As the first systematic collection of archaeological data from a space site outside Earth, this analysis of two areas on the ISS as part of the SQuARE payload has shown that novel insights into material culture use can be obtained, such as the use of wall areas as storage or staging posts between activities, the accretion of objects associated with different functions, and the complexity of using material replacements for gravity. These results enable better space station design and raise new questions that will be addressed through analysis of the remaining four squares.
Supporting information
S1 movie. nasa astronaut kayla barron installs the first square for the sampling quadrangle assemblages research experiment in the japanese experiment module (also known as kibo) on the international space station, january 14, 2022..
She places Kapton tape to mark the square’s upper right corner. Credit: NASA.
https://doi.org/10.1371/journal.pone.0304229.s001
S1 Dataset.
https://doi.org/10.1371/journal.pone.0304229.s002
S2 Dataset.
https://doi.org/10.1371/journal.pone.0304229.s003
S3 Dataset. The image annotations are represented according to sample square in json formatted text files.
The data is available in the ‘SQuARE-notebooks’ repository on Github.com in the ‘data’ subfolder at https://github.com/issarchaeologicalproject/SQuARE-notebooks/tree/main ; archived version of the repository is at Zenodo, DOI: 10.5281/zenodo.10654812 .
https://doi.org/10.1371/journal.pone.0304229.s004
S1 File. The ‘Rocket-Anno’ image annotation software is available on Github at https://github.com/issarchaeologicalproject/MRE-RocketAnno .
The archived version of the repository is at Zenodo, DOI: 10.5281/zenodo.10648399 .
https://doi.org/10.1371/journal.pone.0304229.s005
S2 File. The computational notebooks that process the data json files to reshape the data suitable for basic statistics as well as the computation of the Brainerd-Robinson coefficients of similarity are in the.ipynb notebook format.
The code is available in the ‘SQuARE-notebooks’ repository on Github.com in the ‘notebooks’ subfolder at https://github.com/issarchaeologicalproject/SQuARE-notebooks/tree/main ; archived version of the repository is at Zenodo, DOI: 10.5281/zenodo.10654812 . The software can be run online in the Google Colab environment ( https://colab.research.google.com ) or any system running Jupyter Notebooks ( https://jupyter.org/ ).
https://doi.org/10.1371/journal.pone.0304229.s006
https://doi.org/10.1371/journal.pone.0304229.s007
Acknowledgments
We thank Chapman University’s Office of Research and Sponsored Programs, and especially Dr. Thomas Piechota and Dr. Janeen Hill, for funding the Implementation Partner costs associated with the SQuARE payload. Chapman’s Leatherby Libraries’ Supporting Open Access Research and Scholarship (SOARS) program funded the article processing fee for this publication. Ken Savin and Ken Shields at the ISS National Laboratory gave major support by agreeing to sponsor SQuARE and providing access to ISS NL’s allocation of crew time. David Zuniga and Kryn Ambs at Axiom Space were key collaborators in managing payload logistics. NASA staff and contractors were critical to the experiment’s success, especially Kristen Fortson, Jay Weber, Crissy Canerday, Sierra Wolbert, and Jade Conway. We also gratefully acknowledge the help and resources provided by Dr. Erik Linstead, director of the Machine Learning and Affiliated Technology Lab at Chapman University. Aidan St. P. Walsh corrected the color and lens barrel distortion in all of the SQuARE imagery. Rao Hamza Ali produced charts using accessible color combinations for Figs 3 and 5 . And finally, of course, we are extremely appreciative of the efforts of the five USOS members of the Expedition 66 crew on the ISS—Kayla Barron, Raja Chari, Thomas Marshburn, Matthias Maurer, and Mark Vande Hei—who were the first archaeologists in space.
- 1. Buchli V. Extraterrestrial methods: Towards an ethnography of the ISS. In: Carroll T, Walford A, Walton S, editors. Lineages and advancements in material culture studies: Perspectives from UCL anthropology. London: Routledge; 2021, pp. 17–32.
- 2. Gorman A, Walsh J. Archaeology in a vacuum: obstacles to and solutions for developing a real space archaeology. In: Barnard H, editor. Archaeology outside the box: investigations at the edge of the discipline. Los Angeles, Cotsen Institute of Archaeology Press; 2023. pp. 131–123.
- 3. Walsh J. Adapting to space: The International Space Station Archaeological Project. In: Salazar Sutil JF, Gorman A, editors. Routledge handbook of social studies of outer space. London, Routledge; 2023. pp. 400–412. https://doi.org/10.4324/9781003280507-37
- View Article
- Google Scholar
- 6. Rathje W, Murphy C. Rubbish! The archaeology of garbage Tucson: University of Arizona Press; 2001.
- 7. De León J. The land of open graves: living and dying on the migrant trail. Berkeley, University of California Press; 2015.
- 8. Garrison Darrin A, O’Leary B, editors. Handbook of space engineering, archaeology, and heritage. Boca Raton, CRC Press; 2009.
- 9. Capelotti PJ. The human archaeology of space: Lunar, planetary, and interstellar relics of exploration. Jefferson, NC, McFarland Press; 2010.
- 11. Gorman A. Space and time through material culture: An account of space archaeology. In: Salazar Sutil JF, Gorman A, editors. Routledge handbook of social studies of outer space. London, Routledge; 2023. pp. 44–56. https://doi.org/10.4324/9781003280507-5
- 17. NASA. NASA Johnson. 2008 Aug [cited May 12 2024]. In: Flickr [Internet]. San Francisco. Available from https://www.flickr.com/photos/nasa2explore/
- 19. NASA. ISS Daily Status Reports. 2012 Mar 1 [Cited May 12 2024]. Available from: https://blogs.nasa.gov/stationreport/
- 20. NASA. Man-systems integration. STD-3000 Vol. 1. Houston, NASA Johnson; 1995, pp. 9–15, 78
- 21. NASA. Maintenance Work Area | Glenn Research Center. 2020 Mar 6 [cited May 12 2024]. Available from: https://www1.grc.nasa.gov/space/iss-research/mwa/
- 22. Cristoforetti S. Diario di un’apprendista astronauta. Milan, Le Polene; 2018. pp. 379.
- 23. Kelly S. Endurance: A year in space, a lifetime of discovery. New York, Knopf; 2017. pp. 175, 285–86.
- 24. Barron K. Instagram post, 2022 Feb 12 [cited 2024 May 12]. Available from: https://www.instagram.com/tv/CZ4pW9HJ2Wg/?igsh=ZDE1MWVjZGVmZQ==
- 25. NASA. NASA space flight human-system standard. STD-3001 Volume 1: Human integration design handbook. Rev. 1 Houston, NASA Johnson; 2014. pp. 814, 829–833.
- 27. Keeter B. ISS daily summary report– 2/21/2022. 2022 Feb 21 [cited May 12 2024]. In: NASA ISS On-Orbit Status Report blog [Internet]. Houston. Available from: https://blogs.nasa.gov/stationreport/2022/02/page/6/
- 28. DLR. Fingerprint research to combat harmful bacteria. 2022 Jan 18 [cited May 12 2024]. Available from: https://www.dlr.de/en/latest/news/2022/01/20220118_fingerprint-research-to-combat-harmful-bacteria
- 31. Peeples MA. R script for calculating the Brainerd-Robinson coefficient of similarity and assessing sampling error. 2011 [cited May 12 2024]. Available from: http://www.mattpeeples.net/br.html .
- 33. Garcia M. Cargo Dragon Splashes Down Ending SpaceX CRS-24 Mission. 2022 Jan 24 [cited May 12 2024]. NASA Space Station blog [Internet]. Available from: https://blogs.nasa.gov/spacestation/2022/01/24/cargo-dragon-splashes-down-ending-spacex-crs-24-mission/
- 34. ESA. Concrete Hardening | Cosmic Kiss 360°. 2022 Mar 5 [cited May 12 2024]. Available from: https://www.esa.int/ESA_Multimedia/Videos/2022/05/Concrete_Hardening_Cosmic_Kiss_360
- 35. Keeter B. ISS daily summary report– 2/01/2022. 2022 Feb 1 [cited May 12 2024]. In: NASA ISS On-Orbit Status Report blog [Internet]. Houston. Available from: https://blogs.nasa.gov/stationreport/2022/02/page/19/
- 36. Keeter B. ISS daily summary report– 2/17/2022. 2022 Feb 17 [cited May 12 2024]. In: NASA ISS On-Orbit Status Report blog [Internet]. Houston. Available from: https://blogs.nasa.gov/stationreport/2022/02/page/8/
- 37. T. Pultarova, How Do You Clean a Space Station? Astronaut Thomas Pesquet Shares Orbital Spring Cleaning Tips, Space.com, May 6, 2021. Online at https://www.space.com/space-station-cleaning-tips-astronaut-thomas-pesquet
- 38. Keeter B. ISS daily summary report– 2/22/2022. 2022 Feb 22 [cited May 12 2024]. In: NASA ISS On-Orbit Status Report blog [Internet]. Houston. Available from: https://blogs.nasa.gov/stationreport/2022/02/page/5/
- 39. Keeter B. ISS daily summary report– 2/02/2022. 2022 Feb 2 [cited May 12 2024]. NASA ISS On-Orbit Status Report blog [Internet]. Houston. Online at https://blogs.nasa.gov/stationreport/2022/02/page/18/
- 40. Keeter B. ISS daily summary report– 3/03/2022. 2022 Mar 3 [cited May 12 2024]. In: NASA ISS On-Orbit Status Report blog [Internet]. Houston. Available from: https://blogs.nasa.gov/stationreport/2022/03/page/21/
- 41. Keeter B. ISS daily summary report– 2/08/2022. 2022 Feb 8 [cited May 12 2024]. NASA ISS On-Orbit Status Report blog [Internet]. Houston. Available from: https://blogs.nasa.gov/stationreport/2022/02/page/15/
- 42. Aristotle of Stageira. Metaphysics, Volume I: Books 1–9, Tredennick H, translator. Loeb Classical Library 271. Cambridge, MA, Harvard University Press; 1933. pp. 429–473.
- 44. Hodder I. Entangled: An archaeology of the relationships between humans and things. Hoboken. NJ, Wiley-Blackwell; 2012.
- 45. Malafouris L., How Things Shape the Mind: A Theory of Material Engagement (MIT Press, 2016).
- 46. Keeter B. ISS daily summary report– 3/11/2022. 2022 Mar 11 [cited May 12 2024]. NASA ISS On-Orbit Status Report blog [Internet]. Houston. Available from: https://blogs.nasa.gov/stationreport/2022/03/page/15/
Microgravity research after the International Space Station
Creative Commons
Attribution-NonCommercial-NoDerivatives 4.0 International
Image: Bryce Vickmark
via MIT News
March 12, 2019
- #technology
- Christine Joseph Former Research Affiliate
- Danielle Wood Associate Professor of Media Arts and Sciences; Associate Professor (Joint) of Aeronautics and Astronautics
- Accessibility of the microgravity research ecosystem
Share this article
By Jennifer Chu
For nearly 20 years, the International Space Station (ISS) has served as a singular laboratory for thousands of scientists, students, and startups around the world, who have accessed the station’s microgravity environment to test how being in space impacts everything from cancer cells and human tissues to zucchini and barley seeds — not to mention a host of living organisms including flatworms, ants, geckos, and bobtail squids.
Indeed, the ISS “has operated as a bastion of international cooperation and a unique testbed for microgravity research,” write MIT engineers in a paper they presented on March 8 at the IEEE Aerospace Conference in Montana. But the ISS will eventually be retired in its current form. NASA is preparing to transition the focus of its human space flight activities to the Moon, and the international partners that manage the ISS are discussing how to transition out of the current operational model.
As NASA explores options for commercial entities to operate research platforms in orbit around Earth, and while other public and private entities consider alternative designs for microgravity facilities, the MIT team says it’s important to keep affordable access to such facilities at the forefront of these discussions. In their paper, the researchers argue that scientists from any country should be able to participate in microgravity research.
Toward that end, the team has developed a tool for evaluating the accessibility of various “governance models,” such as facilities that are controlled by mostly governments or private entities, or a mixture of both.
MIT News checked in with the researchers about the future of microgravity research and how openness can drive innovation and collaboration in space. Christine Joseph is a graduate student in MIT’s Department of Aeronautics and Astronautics and the Technology and Policy Program. Danielle Wood is the Benesse Corporation Career Development Assistant Professor of Research in Education within MIT’s Program in Media Arts and Sciences and jointly appointed in the Department of Aeronautics and Astronautics. She is also founder of the Space Enabled Research Group within the MIT Media Lab, whose mission is to advance justice in Earth’s complex systems using designs enabled by space.
Q: Why is affordable access important, particularly for space-based microgravity research?
Wood: Participation in space-based microgravity research should be an opportunity open to researchers from every nation because space is a global commons that does not belong to a single nation. As stated in the Outer Space Treaty, ratified by over 100 countries, “the exploration and use of outer space … shall be carried out for the benefit and in the interests of all countries … and shall be the province of all [hu]mankind.”
Studies in the microgravity environment bring new knowledge about the human body, plants, animals, materials, physics, manufacturing, and medicines. This knowledge can contribute to sustainable development when it is translated into Earth-based applications, such as when knowledge of astronaut exercise routines informs recovery procedures for patients facing long periods of bedrest, or when experiments about the physics of combustion yield results that can improve fire safety on Earth .
When a larger variety of researchers from around the world participate in microgravity research, the scientific community benefits from the broader range of research outcomes. Participation in microgravity research also helps countries that do not yet have experience in space build local capability to design and operate space-based experiments.
Q: How does your new tool evaluate accessibility to microgravity research facilities?
Joseph: We propose that accessibility can be measured using the metrics of economic and administrative openness. Economic openness is based on the financial costs paid by researchers to perform all the activities involved with completing a microgravity research project. This includes the costs associated with designing an experiment, engineering it to be safe and functional, launching it to space, accessing a facility that provides environmental control, data and power, operating the experiment, and possibly returning it to Earth.
Administrative openness refers to the type of gatekeeping that directly and indirectly determines who can participate. For example, today administrative procedures influence access depending on the nationality or type of organization the user comes from and the type of microgravity activity they are seeking. We map future microgravity research facilities and their governance policies along these dimensions of economic and administrative openness. Using these two metrics, we can rate the overall accessibility of a future marketplace for microgravity research.
Wood: Our goal is to encourage a dialogue about the value of providing access to this unique research environment. Many stakeholders — governments, companies, international organizations — may influence the rules that determine who sends micrgravity research to space after the International Space Station is retired. Thus far, the world has not experienced a microgravity research marketplace that is fully driven by commercial forces with prices set by a free market, because governments have subsidized the cost of research access as a public service. This work highlights the need to evaluate future policy and commercial proposals based on the needs of those that have the least access and experience with microgravity research today.
Q: What type of facility or structure have you found, through your tool, can provide the most affordable access to microgravity research, and what will it take to launch such a model?
Joseph: Although not ideal, our current structure has evolved to become surprisingly accessible. Faciliators like the United Nations Office for Outer Space Affairs help to broker access for emerging space nations by working with some of the “gatekeeper” space agencies that built the ISS. Commercial companies have also started to build and operate their own modules attached to the ISS that almost any user can buy access to. The ISS has become this interesting conglomeration of public, private, commercial, and international entities. So far, none of the other proposals for space stations in low Earth orbit (up to about 2,000 kilometers from the Earth’s surface) are mature enough to determine whether they will have a similar level of accessibility as the current environment.
However, we can always do better. Building the ISS was the single largest and most expensive construction project in human history and it involved effort from many countries. There are a lot of lessons to be learned from the development of the ISS in terms of technical and policy models. We also need to take into account the expectations of the commercial companies that will participate in the emerging commercial space economy in low Earth orbit.
The “spaces in space” that we operate in are evolving dramatically. It is not too early to examine how policies and investment decisions will shape the nature of accessibility for microgravity research beyond the International Space Station. Thinking about accessibility now is important to help ensure that microgravity research remains the province of all humankind.
Understanding Socio-Technical Issues Affecting the Current Microgravity Research Marketplace
C. Joseph and D. Wood, "Understanding Socio-Technical Issues Affecting the Current Microgravity Research Marketplace," 2019 IEEE Aerospace Conference, Big Sky, MT, USA, 2019, pp. 1-10. doi: 10.1109/AERO.2019.8742202
Christine Joseph and Danielle Wood co-author "Analysis of the Microgravity Research Ecosystem and Market Drivers of Accessibility"
Christine Joseph and Danielle Wood of Team Space Enabled published a peer reviewed journal paper with the Journal New Space.
Analysis of the Microgravity Research Ecosystem and Market Drivers of Accessibility
Joseph, Christine, and Danielle Wood. "Analysis of the Microgravity Research Ecosystem and Market Drivers of Accessibility." New Space (2021). http://doi.org/10.1089/space.2020.0044
A micro- and macro- analysis of human-machine interfaces and systems in space
Joseph, Christine. "A micro- and macro- analysis of human-machine interfaces and systems in space," September 2020.
Thank you for visiting nature.com. You are using a browser version with limited support for CSS. To obtain the best experience, we recommend you use a more up to date browser (or turn off compatibility mode in Internet Explorer). In the meantime, to ensure continued support, we are displaying the site without styles and JavaScript.
- View all journals
- Explore content
- About the journal
- Publish with us
- Sign up for alerts
- Open access
- Published: 10 November 2020
Space station biomining experiment demonstrates rare earth element extraction in microgravity and Mars gravity
- Charles S. Cockell ORCID: orcid.org/0000-0003-3662-0503 1 na1 ,
- Rosa Santomartino ORCID: orcid.org/0000-0002-8399-7138 1 na1 ,
- Kai Finster ORCID: orcid.org/0000-0002-9132-5542 2 ,
- Annemiek C. Waajen ORCID: orcid.org/0000-0002-2330-1514 1 ,
- Lorna J. Eades 3 ,
- Ralf Moeller 4 ,
- Petra Rettberg ORCID: orcid.org/0000-0003-4439-2395 4 ,
- Felix M. Fuchs 4 , 5 ,
- Rob Van Houdt ORCID: orcid.org/0000-0002-7459-496X 6 ,
- Natalie Leys ORCID: orcid.org/0000-0002-4556-5211 6 ,
- Ilse Coninx 6 ,
- Jason Hatton 7 ,
- Luca Parmitano 7 ,
- Jutta Krause 7 ,
- Andrea Koehler 7 ,
- Nicol Caplin 7 ,
- Lobke Zuijderduijn 7 ,
- Alessandro Mariani 8 ,
- Stefano S. Pellari 8 ,
- Fabrizio Carubia 8 ,
- Giacomo Luciani 8 ,
- Michele Balsamo 8 ,
- Valfredo Zolesi 8 ,
- Natasha Nicholson 1 ,
- Claire-Marie Loudon 1 ,
- Jeannine Doswald-Winkler 9 ,
- Magdalena Herová 9 ,
- Bernd Rattenbacher 9 ,
- Jennifer Wadsworth 10 ,
- R. Craig Everroad 10 &
- René Demets 7
Nature Communications volume 11 , Article number: 5523 ( 2020 ) Cite this article
29k Accesses
70 Citations
1439 Altmetric
Metrics details
- Astrobiology
- Civil engineering
- Industrial microbiology
Microorganisms are employed to mine economically important elements from rocks, including the rare earth elements (REEs), used in electronic industries and alloy production. We carried out a mining experiment on the International Space Station to test hypotheses on the bioleaching of REEs from basaltic rock in microgravity and simulated Mars and Earth gravities using three microorganisms and a purposely designed biomining reactor. Sphingomonas desiccabilis enhanced mean leached concentrations of REEs compared to non-biological controls in all gravity conditions. No significant difference in final yields was observed between gravity conditions, showing the efficacy of the process under different gravity regimens. Bacillus subtilis exhibited a reduction in bioleaching efficacy and Cupriavidus metallidurans showed no difference compared to non-biological controls, showing the microbial specificity of the process, as on Earth. These data demonstrate the potential for space biomining and the principles of a reactor to advance human industry and mining beyond Earth.
Similar content being viewed by others
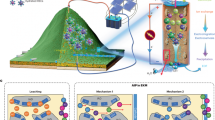
A green and efficient technology to recover rare earth elements from weathering crusts

Microbial growth in actual martian regolith in the form of Mars meteorite EETA79001
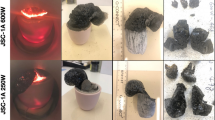
The microstructure and mechanical properties of microwave-heated lunar simulants at different input powers under vacuum
Introduction.
On Earth, microorganisms play prominent roles in natural processes such as the weathering of rocks into soils and the cycling of elements in the biosphere. Microorganisms are also used in diverse industrial and manufacturing processes 1 , 2 , 3 , 4 , for example in the process called biomining (or bioleaching) 5 , 6 . Microorganisms can catalyse the extraction of valuable elements from rocks, such as copper and gold 7 , 8 . This process can in some circumstances reduce the environmentally damaging use of toxic compounds such as cyanides 9 , 10 . These microbial interactions with minerals are also used to decontaminate polluted soils, in a process called bioremediation 10 . Acidophilic iron and sulfur-oxidisers are often used to biomine economic elements from sulfidic ores, but heterotrophic microorganisms, including bacteria and fungi, can be effective in bioleaching in environments with circumneutral or alkaline pH. These organisms can enable leaching by changing the local pH in the environment, for example by the release of protons or organic acids. Alternatively, leaching and sequestration of elements can occur as a consequence of the release of complexing compounds 11 , 12 , 13 , 14 , 15 .
Of important economic and practical interest are rare earth elements (REEs), which include the lanthanides, scandium and yttrium. On account of their physical properties, including ferromagnetism and luminescence, REEs are used in electronic devices such as cell phones and computer screens, as well as in catalysis, metal alloy and magnet production, and many other high-technology applications. Some REEs are identified as short-term near-critical elements 16 , meaning that the demand will soon outstrip supply. Microorganisms are known to be able to mobilise REEs. For example, REEs are used as a cofactor in alcohol dehydrogenases in diverse microbial taxa 17 , 18 , and they were shown to be essential for the survival of an acidophilic methanotroph in a volcanic mudpot 19 . The ability of microorganisms to mobilise REEs from rocks has been shown for a variety of different mineral matrices 20 , 21 .
As humans explore and potentially settle in space, microbe–mineral interactions have been recognised to be important, including in biomining 22 , 23 , 24 . In addition to mining beyond the Earth, advancing our understanding of microbe–mineral interactions in space could be applied to: (1) soil formation from nutrient-poor rocks 22 , (2) formation of biocrusts to control dust and surface material in enclosed pressurised spaces 25 , (3) use of regolith as feedstock within microbial segments of life support systems 26 , (4) use of regolith and microbes in microbial fuel cells (biofuel) 22 , (5) biological production of mineral construction materials 27 . All of these diverse applications have in common that they require experimental investigations on how microbes attach to, and interact with, rock and regolith materials in space environments. Furthermore, there is a need to know how organisms alter ion leaching and mineral degradation in altered gravity regimens, which will occur in any extraterrestrial location.
Altered gravity conditions, such as microgravity, are known to influence microbial growth and metabolic processes 28 , 29 , 30 . Although the capacity of prokaryotes to directly sense gravity remains a point of discussion, gravity influences sedimentation and convection in bulk fluids 31 . By allowing for thermal convection and sedimentation, gravity is thought to affect the mixing of nutrients and waste, thereby influencing microbial growth and metabolism 32 , 33 , 34 , 35 . Based on these considerations, we hypothesised that altered gravity regimens would induce changes in microbial interactions with minerals, and thus bioleaching.
In this work, we present the results of the European Space Agency BioRock experiment, performed on the International Space Station (ISS) in 2019 to investigate the leaching of elements from basalt 36 , 37 , 38 , an analogue for much of the regolith material on the Moon and Mars, by three species of heterotrophic microorganisms. The experiment compared bioleaching at three different levels of gravity: microgravity, simulated Mars and terrestrial gravity. Results are reported on the bioleaching of REEs, demonstrating the effective use of microorganisms in biomining beyond Earth using a miniaturised space biomining reactor.
REE biomining in space
Data were acquired using the BioRock biomining reactor, designed for these experiments (Fig. 1 ) which contained basaltic rock with known REE composition (Table 1 ) and major elements (Supplementary Table 1 ). REEs bioleached into solution were measured for all three organisms ( S. desiccabilis , B. subtilis , C. metallidurans ) in all three gravity conditions (microgravity, simulated Mars and Earth gravity) and for non-biological controls (Fig. 2 , Supplementary Fig. 1 and Supplementary Table 2 ). The concentrations of leached REEs in biological and non-biological condition generally followed the trends expected from their abundance in the basaltic rock (Table 1 ; Supplementary Table 2 ). Elements with the highest abundance ( e.g . Ce and Nd) showed the highest leached concentrations while elements with lowest abundance (Tb, Tm and Lu) exhibited the lowest concentrations.
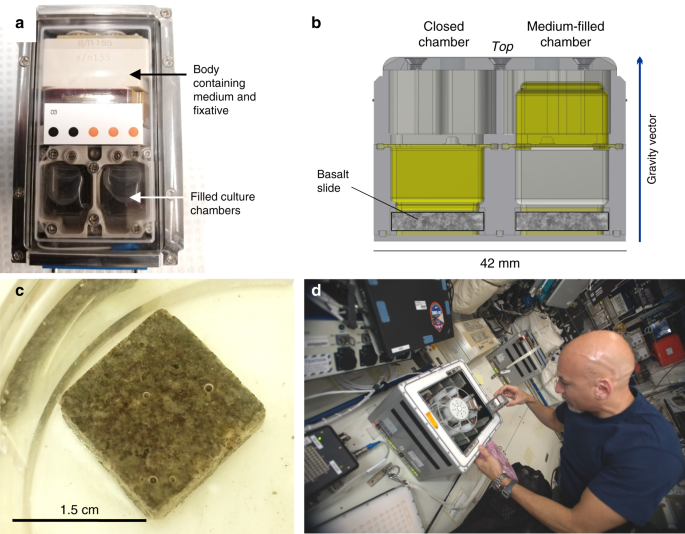
a Top-down image of one Experimental Container (EC) containing one EU (Experimental Unit) showing both culture chambers inflated with medium. b Sideways cross section through culture chamber showing location of basalt slide at the back of the chamber and principle of medium injection and inversion of membrane (shown here in yellow; left side closed, right side inflated with medium). c Image of basalt slide in a Petri dish submerged in 50% R2A in a ground experiment. d ESA astronaut Luca Parmitano inserts an EC into a KUBIK incubator on board the International Space Station (image credit to ESA).
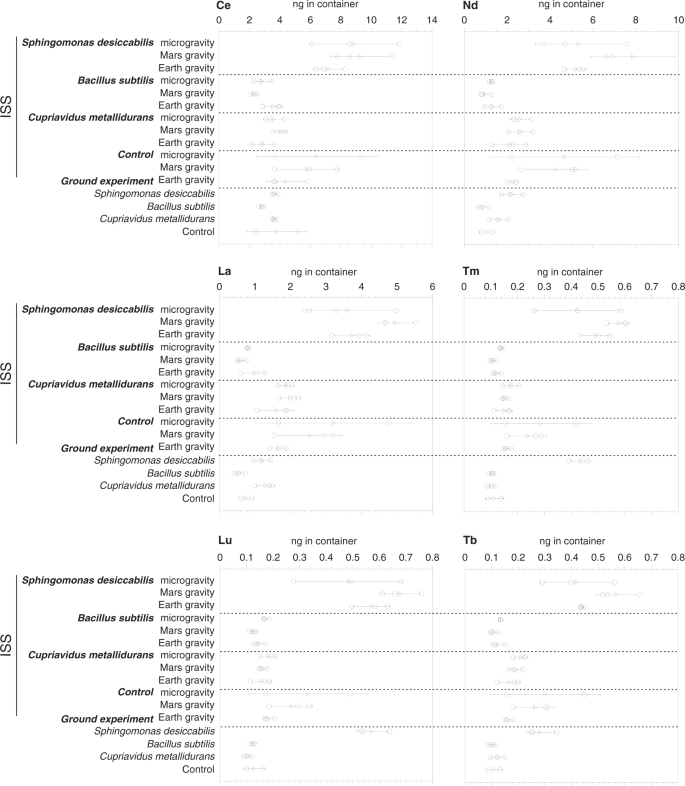
Concentrations (ng in total chamber liquid) of rare earth elements (REEs) in each of the experimental flight and ground control samples at the end of the experiment (described in the text) for each of the three organisms and non-biological controls. The three most (Ce, Nd, La) and least (Tm, Lu, Tb) abundant REEs are shown here (all others in Supplemental Fig. 1 ). ISS shows the International Space Station flight experiments. Circles show triplicate measurements (n = 3 biologically independent samples. One non-biological microgravity and non-biological ground control sample were lost and are not shown) and the mean is given as a triangle. Error bars represent standard deviations.
Statistical analysis across all three organisms and the three gravity conditions tested in space showed a significant effect of the organism (ANOVA: F (2,369) = 87.84, p = 0.001) on bioleaching. Post-hoc Tukey tests showed all pairwise comparisons between organisms to be significant ( p < 0.001). There was a non-significant effect when gravity conditions were compared (ANOVA: F (2,369) = 0.202, p = 0.818). The interaction between gravity and the organism was not significant (ANOVA: F (4, 369) = 1.75, p = 0.138).
Statistical analysis was carried out on S. desiccabilis bioleaching. Comparing the difference between biological samples and the non-biological controls in each gravity condition for S. desiccabilis showed that microgravity was not significant (ANOVA: F (1,69) = 2.43, p = 0.124), but significant differences between the biological experiments and the non-biological controls were observed in simulated Mars (ANOVA: F (1,83) = 14.14, p < 0.0001) and Earth gravity (ANOVA: F (1,83) = 24.20, p < 0.0001). The difference in bioleaching between gravity conditions was not significant (ANOVA: F (2,123) = 1.60, p = 0.206) for S. desiccabilis .
For S. desiccabilis , across all individual REEs and across all three gravity conditions on the ISS, the organism had leached 111.9% to 429.2% of the non-biological controls (Fig. 3a and Supplementary Table 3 ). Student’s t tests were used to examine the concentration of individual REEs bioleached compared to non-biological controls. Bioleaching was significantly higher than non-biological controls under simulated Mars and Earth gravity for individual REEs ( p < 0.05, Student’s t test, Supplementary Table 4 ), except for Pr and Nd which were significantly higher at the p < 0.1 level, and not significant for Ce in simulated Mars gravity ( p = 0.102). For the microgravity condition, none of individual REE concentrations in the biological experiment was significantly higher than the non-biological control ( p > 0.05) (Supplementary Table 4 ). The standard deviations of the microgravity biological and non-biological controls for the individual REEs for S. desiccabilis were, apart from Pr in the biological experiment, higher than for B. subtilis and C. metallidurans .
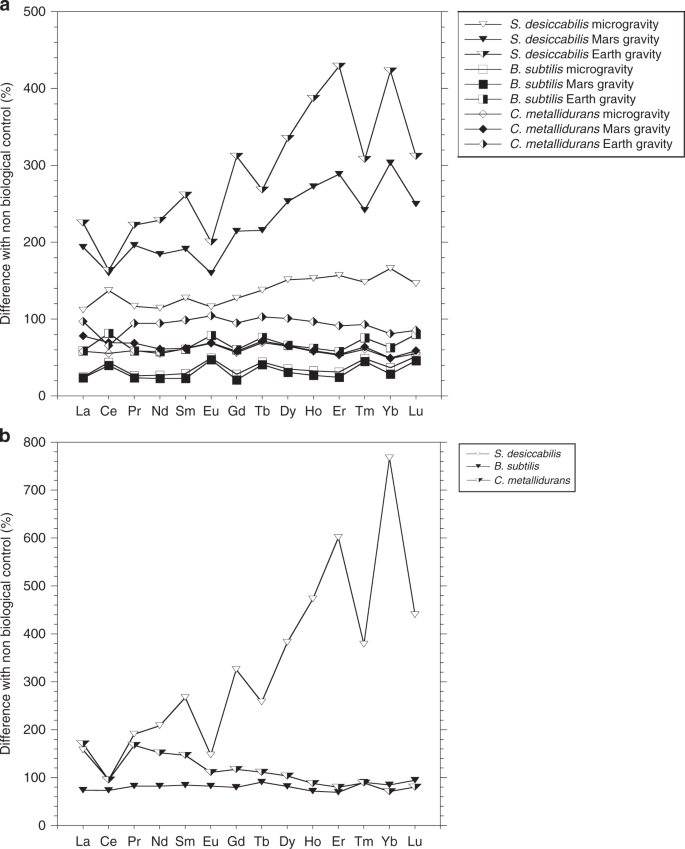
a Relative (%) difference in mean concentration of leached REEs in the bulk fluid between biological experiments and non-biological controls showing microgravity, simulated Mars and Earth gravities on the International Space Station for the three microorganisms. b Ground (true Earth gravity control) experiment for the three microorganisms. Standard deviations reported in Supplemental Table 3 , statistics reported in the main text.
Student’s t test comparisons were carried out between the concentrations of bioleached REEs in different gravities for each element for S. desiccabilis (Supplementary Table 4 ). Comparison between the simulated Mars gravity and simulated Earth gravity showed that the concentrations of five elements (La, Sm, Eu, Tb, Ho) were significantly different at the p < 0.05 level and five more elements (Gd, Dy, Er, Tm, Yb) at the p < 0.1 level, with simulated Earth gravity values being higher. These differences were more evident among the ‘heavy’ REEs (elements from Gd up to Lu) (Fig. 3a ). The total quantity of REEs released by S. desiccabilis as a percentage of the available quantity in the basalt, ranged between 1.17 × 10 −1 and 2.41 × 10 −2 % (Supplementary Table 5 ).
Identical statistical analysis was carried out for bioleaching experiments with B. subtilis and C. metallidurans . For B. subtilis , the quantity of REEs bioleached was significantly less than the non-biological controls in microgravity (ANOVA: F (1,69) = 13.05, p < 0.001) and simulated Mars gravity (ANOVA: F (1,83) = 29.55, p < 0.0001), but marginally not significant in Earth gravity (ANOVA: F (1,83) = 3.79, p = 0.055). The difference in the concentrations of REEs bioleached between gravity conditions was not significant (ANOVA: F (2,123) = 1.45, p = 0.240).
For C. metallidurans , the difference between bioleaching and the non-biological controls was not significant in all three gravity conditions: microgravity (ANOVA: F (1,69) = 2.25, p < 0.138), simulated Mars (ANOVA: F (1,83) = 3.47, p < 0.066), and Earth gravity (ANOVA: F (1,83) = 0.265, p = 0.608). The difference in bioleaching between gravity conditions was not significant (ANOVA: F (2,123) = 0.71, p = 0.496).
Comparisons were made for each REE leached into solution in the biological experiments compared to the non-biological control for B. subtilis and C. metallidurans and for each separate gravity condition ( t -test). In B. subtilis , for simulated Mars and Earth gravity, concentrations of bioleached REEs in solution were significantly lower compared to the non-biological control (Supplementary Table 4 ) at the p < 0.05 level, except for Eu, Gd, Tb, Ho and Lu, which were significantly lower at the p < 0.1 level, and not significant for Ce in the simulated Earth gravity condition ( p value = 0.378). In C. metallidurans , Tm, Yb and Lu were statistically lower at the p < 0.1 level in simulated Mars gravity (Supplementary Table 4 ).
Comparisons were also made for each REE leached into solution in the biological experiments between gravity conditions for B. subtilis and C. metallidurans ( t -test). In B. subtilis cultures, six elements (Dy, Ho, Er, Tm, Yb, Lu) showed a difference at the p < 0.05 level between microgravity and simulated Mars gravity and one element (Ce) at the p < 0.05 level between simulated Mars and Earth gravity. For C. metallidurans cultures, Ce was the only element that showed a significant difference at the p < 0.01 level between microgravity and simulated Mars gravity. For both B. subtilis and C. metallidurans , concentrations of elements leached as a percentage of the total available in the basalt ranged from 3.22 × 10 −2 to 4.14 × 10 −3 % (Supplementary Table 5 ).
To test whether the REEs were absorbed onto the cell membrane or within the microbial cell, ICP-MS analyses of the cell pellets were performed (Supplementary Table 6 ). The concentrations of REEs in these samples generally accounted for less than 5% of the total REEs in the bulk solution in the biological experiments, with a few exceptions. Notably, Eu was above 5% in all conditions apart from S. desiccabilis in microgravity and Mars gravity. ANOVA was used to ascertain whether the biological enhancement of REEs leached into solution exhibited by S. desiccabilis was also reflected in the quantity of REEs bound to cells compared to the two other organisms. In microgravity, there was a significant difference between the organisms (ANOVA: F (2,125) = 3.98, p = 0.021), but post-hoc Tukey showed that only the S. desiccabilis and B. subtilis pairwise comparison was significant ( p = 0.016). There was no significant difference between organisms in Mars gravity (ANOVA: F (2,125) = 0.466, p = 0.629). In Earth gravity, there was a significant difference (ANOVA: F (2,125) = 36.94, p < 0.001) with post-hoc Tukey showing p < 0.001 for all pairwise comparisons apart from S. desiccabilis and C. metallidurans ( p = 0.132). In almost all cases the percentage of total REEs associated with the S. desiccabilis cell pellets were lower than the other two organisms (Supplementary Table 6 ). Thus, there was no evidence for a systematically higher fraction of REEs in the S. desiccabilis cell pellets. The concentrations of REEs in the supernatant produced from washing of the cell pellet was below the detection limit.
Comparison of the REEs leached into solution between the different gravity regimens of the non-biological control samples on the ISS (Figs. 2 , 3a , Supplementary Fig. 1 and Supplementary Table 2 ) showed that the gravity condition was not significant (ANOVA: F (2,109) = 2.91, p = 0.059). Student’s t test investigations of individual elements in each gravity condition (Supplementary Table 4 ) showed that Pr, Nd, Sm, Eu, Gd, Tb, Dy were significantly different (at the p < 0.1 level) between simulated Mars and Earth gravity control samples. The pure 50% R2A medium and NOTOXhisto fixative contributed low concentrations of REEs (<0.1 ng to the total solution concentration).
S. desiccabilis caused preferential leaching of heavy REEs
The percentage difference in bioleaching of REEs was calculated for each microorganism relative to the leaching in the non-biological controls in the same gravity condition, for space and ground experiments (Fig. 3 and Supplementary Table 3 ).
S. desiccabilis caused preferential leaching of heavy (Gd up to Lu) over light (La up to Eu) REEs. On the ISS, the highest enhancement was a 429.2 ± 92.0% increase in Er leaching in simulated Earth gravity, compared to the non-biological control. On the ground, Yb showed the highest enhancement of 767.4 ± 482.4% increase in bioleaching over the non-biological control. The larger differences between the non-biological and the biological leaching of heavy REEs compared to light REEs is reflected in generally lower p values (Student’s t -tests) for heavy REEs compared to light REEs (Supplementary Table 4 ).
Performance of biomining in space and true Earth gravity
In parallel with the ISS experiment, ground experiments (true Earth gravity control) were conducted. Results from the ground control experiments are reported in Figs. 2 , 3b , Supplementary Fig. 1 , Supplementary Tables 2 and 3 . For S. desiccabilis , the effect of the microorganism on leaching in the ground control compared with the non-biological control was significant (ANOVA: F (1,68) = 24.56, p < 0.001). All individual elements showed a statistically significant difference (Student’s t test) with the non-biological control (Supplementary Table 4 ) at the p < 0.05 level apart from two elements at the p < 0.1 level (Nd and Sm) and three elements with no significant difference (La, Ce, Eu). For B. subtilis , the effect of the microorganism on leaching was not significant (ANOVA: F (1,68) = 0.034, p = 0.854), similarly with C. metallidurans (ANOVA: F (1,68) = 0.705, p = 0.404).
Bioleaching of REEs in simulated Earth gravity on the ISS was compared to bioleaching in the ground experiment (true Earth gravity). S. desiccabilis showed a significant difference (ANOVA: F (1,82) = 8.14, p = 0.005) with simulated Earth gravity on ISS being higher across all REEs. Neither B. subtilis (ANOVA: F (1,82) = 2.42, p = 0.124) or C. metallidurans (ANOVA: F (1,82) = 2.45, p = 0.121) showed a significant difference. Non-biological controls exhibited a significant difference between the simulated Earth gravity on the ISS and ground controls (ANOVA: F (1,68) = 6.90, p = 0.011) with the concentration of REEs leached into solution in simulated Earth gravity on the ISS being higher across all REEs.
Biomining occurred under near neutral pH conditions
The pH status is an important factor in the efficacy of biomining. NOTOXhisto fixative lowers the final pH of the solutions, so that the pH at the end of the experiment is not representative of the pH during growth. In all experimental solutions, the final pH ranged between 4.16 ± 0.20 and 6.12 ± 0.01 (Supplementary Table 7 ).
As it was not possible to measure the pH during the experiment on the ISS, a ground experiment was conducted to investigate pH changes over the 21 days of growth at 20–22 °C. Results are shown in Supplementary Fig. 2 . The pH remained circumneutral for the non-biological samples throughout the experiment with slight differences in the presence of basalt. The presence of bacteria caused the pH to rise during the 21 days compared to the negative controls, regardless of the specific species. At day 21, the pH values for the three cultures in the presence of basalt were: S. desiccabilis , 8.41 ± 0.01; B. subtilis , 8.63 ± 0.01; C. metallidurans , 8.66 ± 0.01, and the non-biological control 7.35 ± 0.036 (mean ± sd). The presence of the basalt slide caused slight pH differences within the biological samples during the first week of growth. After one week, the pH remained constant until the end of the experiment for all the microorganisms. After 21 days of growth, the pH values with and without the presence of the rock are similar for each microorganism, suggesting that the influence of the rock material on the pH values stabilised over time (Supplementary Fig. 2 ). There was a large drop in pH after the addition of the fixative ( S. desiccabilis , 3.58 ± 0.07; B. subtilis , 3.89 ± 0.10; C. metallidurans , 3.76 ± 0.08, and the non-biological control 3.08 ± 0.03, mean ± sd). The post-fixative pH values are different depending on the organism, but independent of the presence of the basalt. After one week of cold storage, the presence of the basalt slide caused an increase in pH for all biotic and non-biological samples, indicating that the pH measured in the flight and ground control samples was influenced by both the presence of the basalt slide and the fixative.
This study investigated the use of microorganisms to extract a group of economically important elements (fourteen REEs) from basalt rock, a material found on the Moon and Mars 36 , 37 , 38 , under simulated Mars and Earth gravity on the International Space Station (ISS). Microgravity was investigated as the lowest gravity level possible to explore the effects of a lack of sedimentation on bioleaching, to understand the role of gravity in influencing microbe–mineral interactions in general, and to gain insights into industrial biomining on asteroids and other very low gravity planetary objects. A true Earth gravity ground control experiment was also performed.
The presence of the bacterium S. desiccabilis was found to enhance mean concentrations of leached REEs in all gravity conditions investigated and these enhancements were significant in simulated Mars and Earth gravity on ISS compared to the non-biological controls. Although the S. desiccabilis microgravity samples reached higher mean concentrations than the microgravity non-biological controls for all REEs, the difference was not statistically significant. The statistical result is interpreted to be caused by the greater standard deviations in the leached concentrations of elements in the microgravity biological experiment and non-biological controls and the loss of one of the microgravity control samples owing to contamination, rather than an effect of microgravity on biological leaching.
The lack of a significant difference in the final concentrations of REEs leached by S. desiccabilis when the different gravity conditions were compared is surprising since microgravity has been reported to influence microbial processes 39 , 40 . However, the results are consistent with our observation that final cell concentrations did not differ between the different gravity conditions in the three microorganisms 31 . One reason for the lack of statistically significant differences in final concentrations of REEs between gravity conditions might be that the bacterial cultures had sufficient nutrients to reach their maximum cell concentration 31 , regardless of the different sedimentation rates in each gravity, thus achieving similar leaching concentrations. Hence, the experiments showed that, with the appropriate nutrients, biomining is in principle achievable under a wide range of gravity conditions.
The mechanism for the REE bioleaching in Sphingomonas desiccabilis is unknown. It was not caused by bulk acidification of the growth medium, since the ground experiments showed that the medium had a slightly basic pH profile during the experiment. The microorganism is a prolific producer of extracellular polysaccharide (EPS) and these compounds are known to enhance bioleaching in other organisms by complexing ions in EPS moieties such as uronic acid 41 , 42 . A greater biological enhancement in the leaching of heavy compared to light REEs was observed, a pattern consistent with observations by Takahashi et al. 43 in laboratory cell cultures and natural microbial biofilms. The authors suggested that phosphate moieties on the cell or EPS might preferentially bind heavy REEs, a distinct property of these biologically produced materials. We also note that the authors suggested that heavy REE enrichments could potentially be used as a biosignature for the activities of life. Beyond applications to biomining, our experiments showed the preferential enhancement of heavy REEs in the liquid phase including in simulated Martian gravity, indicating the production of a potential biosignature under altered gravity, with implications for example for additional methods to test the hypothesis of life on Mars.
Enhanced REEs associated with pelleted S. desiccabilis cells compared to the other two species was not observed. The reduced pH caused during fixation and sample preparation may have unbound any REEs attached to cell surfaces in all three species. Alternatively, the majority of the REEs may have bound to the extracellular EPS or have been released directly into solution. We have observed S. desiccabilis by confocal microscopy to form biofilms on the surfaces and at the edges of cavities on the basalt more pervasively than B. subtilis and C. metallidurans under these growth conditions, which could have enhanced cell-mineral interactions and thus leaching of REEs into solution. The analysis of REEs within biofilms did not form part of this study since we wished to separately examine the biofilms non-destructively.
Unavoidable in this experiment was the potential for continued leaching after fixation and during storage, when the pH was reduced in the chamber. However, during storage, the temperature was kept at 2.1 °C on the ISS and below 7.1 °C during sample return to reduce leaching activity 44 . Furthermore, a similar reduction of the pH occurred in the non-biological control samples.
In contrast to S. desiccabilis , B. subtilis demonstrated less mean leaching in the biological experiments than the non-biological controls in all three gravity conditions. This cannot be attributed to cells attached to the rock retarding ion release since the microorganisms did not form substantial biofilms on the surface of the rock and the final cell biomass was lower than in the case of S. desiccabilis 31 . As the pH was likely to be similar to the other organisms during the course of the experiment as shown by our ground-based post-flight pH experiment, differences in pH during the experimental phase cannot explain the results. An alternative explanation could be a chemical effect of cell exudates, such as ligands that retarded leaching or the solubility of REEs. However, despite its previously demonstrated bioleaching activity 45 , 46 , and cell wall absorption of REEs 47 , Kucuker et al. 48 showed that B. subtilis was not able to extract tantalum, a transition metal considered similar to a REE, from capacitors.
C. metallidurans did not enhance leaching of REEs. In a 3-month preparatory phase for the BioRock experiments, the leaching of elements from crushed basalt by this organism on the Russian FOTON-M4 capsule was investigated 49 . In this experiment, C. metallidurans enhanced copper ion release, but other rock elements did not show significantly enhanced leaching. Although the microorganism was suspended in mineral water, the results are consistent with those reported here.
In none of the experiments was a cerium anomaly 50 observed. Unlike other REEs that are all trivalent, cerium can be oxidised to the less soluble Ce 4+ state, which can cause differences in precipitation and concentration compared to other REEs. The experiments were performed under oxic conditions. However, once the cerium was leached from the rock, its oxidation state would not necessarily have changed its presence in the bulk fluid, potentially explaining the lack of an anomaly.
Comparing the Earth gravity simulation on the ISS with the ground-based experiments (true 1 × g control), no significant difference was observed between biological experiments with B. subtilis and C. metallidurans , but there was a significant difference between the S. desiccabilis biological experiments and between the non-biological controls, with ground-based leaching significantly less in some REEs compared to the Earth gravity simulation on the ISS. Simulated gravity in space is not exactly the same as 1 × g on Earth as shear forces induced by centrifugation in space can create different physical conditions. Furthermore, because of the small radius of the centrifuge rotor in KUBIK, gravity forces vary across the culture chamber. We also note that the ground experiment had a 0.46 °C higher temperature offset than the KUBIKs on the ISS during the main experimental phase. The experiment on the ISS involves the launch and download to Earth of the samples, which could influence them in ways that cannot be easily predicted. Nevertheless, the general trends observed in Earth gravity experiments with respect to biologically enhanced leaching for the three organisms were conserved in space.
Our experiment has several differences with any proposed large-scale biomining activity. The basalt rock was not crushed in order to investigate biofilm formation on a flat, contiguous but porous rock surface, another main goal of the BioRock experiment. This may have influenced the total percentage of REEs extracted from the rock, which was generally less than 5 × 10 −2 %. These leaching rates would likely be higher with crushed rocks, which on Earth have been shown to result in leaching efficiencies of REEs of 8.0 × 10 −3 % to several tens of percent under optimised conditions 51 , 52 . Furthermore, we did not stir our reactors as we wanted to investigate the effects of microgravity and Mars gravity on cell growth in the absence of artificial mixing. Understanding which parameters would require adjustments to enhance the process as well as upscaling of the reactor would be the next step. Our experiment demonstrates that the leaching capacities of the three different microorganisms on the Earth 53 , 54 were similar in space. Thus, Earth-based ground experiments provide reliable insights into the biomining capacities of specific organisms in space. Yet, our experiments also confirm that it is important to be careful in the selection of microorganisms for space biomining operations.
Basaltic material was investigated because it is common on the Moon and Mars 36 , 37 , 38 . Our experiment suggests that other materials could return even higher yields. For example, lunar KREEP rocks have unusually high concentrations of REEs 55 , 56 . We did not test lunar gravity (0.16 × g ) directly, but it lies between microgravity and Mars gravity. Our results therefore likely reflect the potential efficacy of biomining operations under lunar gravity. We suggest the construction of REE biomining facilities in the Oceanus Procellarum and Mare Imbrium regions of the Moon, where KREEP rocks are abundant. The principle we demonstrate could be applied to other materials of economic importance for In-Situ Resource Utilisation (ISRU). For example, meteoritic material has been shown to be compatible with microbial growth 26 , 57 , 58 , 59 , 60 and thus our microgravity experiments show the potential for biomining in low gravity asteroid environments.
In conclusion, our results demonstrate the biological mining of economically important elements in space, specifically REEs and in different extraterrestrial gravity environments. The experiments also demonstrate the novel REE bioleaching ability for the mesophilic, biofilm-forming, and desiccation-resistant bacterium S. desiccabilis , which could be used in biomining applications. From a technical point of view, our experiment also demonstrated the principles of a miniature space biomining reactor. The experiment thus shows the efficacy of microbe–mineral interactions for advancing the establishment of a self-sustaining permanent human presence beyond the Earth and the technical means to do that.
BioRock experiment
BioRock was an experiment proposed to European Space Agency (ESA) in response to the International Life Science Research Announcement in 2009 (ILSRA-2009). The project was selected as a candidate for flight in 2010 and subsequent bioreactor hardware design has been described 61 . The experiment began on the International Space Station on July 30, 2019 and ended on August 20, 2019.
Microorganisms and growth media
Three bacterial species were used to investigate bioleaching. Criteria were: (1) they could tolerate desiccation required for experiment preparation, (2) they could grow on solid surfaces and/or form biofilms, (3) they were able to interact with rock surfaces and/or bioleach, and (4) they all could be grown in an identical medium at the same experimental conditions to allow for comparisons between organisms.
The microorganisms used were:
Sphingomonas desiccabilis CP1D (DSM 16792; Type strain), a Gram-negative, non-motile, desiccation resistant, non-spore-forming bacterium, which was isolated from soil crusts in the Colorado plateau 62 .
Bacillus subtilis NCIB 3610 (DSM 10; Type strain), a Gram-positive, motile, spore- and biofilm-forming bacterium naturally found in a range of environments, including rocks 63 . The organism has been used in several space experiments 28 , 33 .
Cupriavidus metallidurans CH34 (DSM 2839; Type strain), a Gram-negative, motile, non-spore forming bacterium. Strains of this species have been isolated from metal-contaminated and rock environments 64 , 65 , 66 , 67 , 68 , 69 . The organism has been previously used in space experiments 70 .
The medium used for the BioRock experiment was R2A 71 at 50% concentration as it supported growth of all three microorganisms 61 , allowing for comparisons. The composition was (g L −1 ): yeast extract, 0.25; peptone, 0.25; casamino acids, 0.25; glucose, 0.25; soluble starch, 0.25; Na-pyruvate, 0.15; K 2 HPO 4 , 0.15; MgSO 4 .7H 2 O, 0.025 at pH 7.2.
NOTOXhisto (Scientific Device Laboratory, IL, USA), a fixative compatible with safety requirements on the International Space Station (ISS), was used to halt bacterial metabolism at the end of the experiment.
Bioleaching substrate
Basalt was used for bioleaching, whose REE composition, as determined by ICP-MS (inductively coupled plasma mass spectrometry) and bulk composition, as determined by X-ray Fluorescence (XRF), is shown in Table 1 and Supplementary Table 1 , respectively. The material was an olivine basalt rock collected near Gufunes, Reykjavik in Iceland (64°08′22.18′′N, 21°47′21.27′′W) chosen because it has a chemical composition similar to that of basalts found on the Moon and Mars 36 , 37 , 38 . The rock was cut into slides of 1.5 cm × 1.6 cm and 3 mm thick. The mass of 15 of these slides was 1.871 ± 0.062 g (mean ± standard deviation). The rock was not crushed, as might be carried out for large-scale bioleaching, because the BioRock project was also concerned with quantifying the formation of microbial biofilms over a contiguous mineral surface.
Sample preparation for flight
The basalt rock slides were sterilised by dry-heat sterilisation in a hot air oven (Carbolite Type 301, UK) for 4 h at 250 °C. This treatment did not change the mineralogy of the rocks as determined by X-Ray Diffraction (XRD).
Single strain cultures of each organism were desiccated on the slides as follows:
S. desiccabilis . An overnight culture of the strain was grown in R2A 100% at 20–22 °C until reaching stationary phase (OD600 = 0.88 ± 0.09; approximately 10 9 colony forming units per mL). Then, 1 mL of the culture was inoculated on each basalt slide and the samples were air-dried at room temperature (≈20–25 °C) with a sterile procedure within a laminar flow-hood.
B. subtilis . Spores were produced as described previously 72 . For each basalt slide, 10 µL of a ≈ 1 × 10 8 spores/mL solution were used as inoculum, i.e. 1 × 10 6 spores per slide, and air-dried at room temperature (≈20–25 °C) within a laminar flow-hood.
C. metallidurans . Samples were prepared using a freeze-dry protocol (Belgian Co-ordinated Collection of Microorganisms, BCCM). Cells were cultured on solid Tryptone Soya Agar (TSA, Oxoid CM0131, BCCM) medium. When grown confluently, cells were harvested with a cotton swab and suspended in cryoprotectant, consisting of sterile horse serum supplemented with 7.5% trehalose and broth medium no. 2 (625 mg L −1 ; Oxoid CM0131, BCCM). Thirty millilitres of bacterial suspension were transferred to a 90 mm petri dish and basalt slides were submerged in the bacterial suspension and gently shaken overnight. Basalt slides, each containing approximately 10 9 colony forming units per mL, were then transferred to a 6-well plate (1 slide per well) and covered with a gas permeable seal and inserted on a pre-cooled shelf of −50 °C, followed by a freezing phase for 90 min at a shelf temperature of −50 °C. Primary drying was performed with a shelf temperature of −18 °C and chamber pressure of 400 mTorr. A secondary drying was performed with a shelf temperature of 20 °C and a chamber pressure below 10 mTorr. After freeze-drying, the 6-well plate was covered with a lid and wrapped in parafilm.
Negative controls were sterile basalt slides without cell inoculation.
After preparation, all samples were stored at room temperature (20–25 °C) until integration in the culture chambers in the bioreactor.
Flight experimental setup
The hardware design, assembly and filling procedure were described previously 61 . Each Experiment Unit (EU) of the BioRock apparatus was designed to accommodate two independent basalt slides in two independent sample chambers (Fig. 1 ). Each EU contained culture medium and fixative reservoirs (Fig. 1a ). To allow oxygen diffusion without contaminating the cultures, each chamber was equipped with a deformable, gas permeable, silicone membrane (Fig. 1b, c ) 61 . After integration of the basalt slides, the medium and fixative reservoirs were filled with 5 mL of medium and 1 mL of fixative for each sample, respectively. The culture chambers and surrounding ducts were purged with ultrapure sterile N 2 gas.
All the samples were integrated under strict aseptic procedures into the EUs. There were 36 samples in 18 EUs for the flight experiment, and 12 samples in 6 EUs for the ground experiment. The EUs were integrated into a secondary container that provided the required two-level containment of the fixative (Fig. 1a ). The EU within the container is referred to as the Experiment Container (EC).
After integration, 18 flight ECs were stored at room temperature (≈20–25 °C) for 2 days. The ECs were launched to the ISS on board of a Space X Dragon capsule, Falcon-9 rocket during CRS-18 (Commercial Resupply Services) mission on July 25, 2019 from the NASA Kennedy Space Center, Cape Canaveral, Florida. On arrival at the ISS, ECs were stored on-board at 2.1 °C.
On the day of the start of the experiment (July 30, 2019), the ECs were installed by astronaut Luca Parmitano into two KUBIK facility incubators, pre-conditioned to a temperature of 20 °C (Fig. 1d ). Medium injection was performed robotically, triggered by internal clocks built within the ECs, powered by electricity provided by the KUBIK incubator. Thereafter the astronaut removed the ECs and took photographs of all culture chambers to obtain evidence of the medium supply and to allow comparison with the same chamber after the experimental growth period. After image acquisition, the ECs were plugged back into the KUBIKs. Two KUBIK incubators were used for BioRock, running in parallel: One was set to simulate Earth gravity (1 × g = 9.81 m/s 2 ) at the surface of the basalt slide where bioleaching is occurring, while the second was set to simulate Mars gravity (0.4 × g = 3.71 m/s 2 ; Mars gravity is strictly 0.38 × g , but finer g resolution is not possible to set in the KUBIK) at the surface of the basalt slide. Gravity levels were measured every 10 min during the active experimental phase using an accelerometer (ADXL313, Analog Devices) mounted on a printed circuit board fixed to the bottom of the EC. The distance between the top face of the basalt slide and the plane of the top face of the PCB was 10.3 mm. A correction factor was applied to account for the longer rotation radius at the basalt slide. These accelerometer (gravity) values are shown in Supplementary Table 8 . The microgravity-exposed ECs were split equally between both KUBIKs and inserted in the static slots available in the facility. The experiment was run for 21 days.
To stop the cultures from growing, the fixative was automatically injected into the culture chambers on August 20, 2019. The samples were removed from the KUBIK incubators and images of the culture chambers were taken. Afterwards, the ECs were stored in refrigeration as described below.
Temperature during space experiment
The temperature of the ECs from pre-flight until post-flight was measured using temperature loggers (Signatrol SL52T sensors, Signatrol, UK) on the rear of four of the ECs. These data showed that temperatures did not exceed 7.1 °C from pre-flight handover until storage after arrival at the ISS. During on-board storage, both before and after the 21-day period of culturing, temperatures were constant at 2.1 °C. During culturing, the loggers recorded a temperature of 20.16 °C in both KUBIKs. The ECs were downloaded from the ISS, packed in a ‘double coldbag’ provided by NASA. Splashdown occurred in the Pacific Ocean on August 27 and handover of the ECs to the investigators occurred on August 29 at Long Beach Airport, LA, USA. Between removal from storage on August 26 on ISS and handover to the science team on August 29, the temperature loggers recorded a temperature of 6.6 °C, rising transiently to 7.1 °C. The ECs were stored in a refrigerated insulated box and transferred to the NASA Ames Research Centre for sample removal on August 30.
Ground experiment
Parallel to the experiment occurring on the ISS, a 1 × g ground experiment (true Earth gravity) was run to compare with the ISS simulated Earth gravity samples. Six ECs for the ground experiment were shipped from the NASA Kennedy Space Center to the NASA Ames Research Centre under cooled (4 °C) conditions. The six ECs were attached to a power system (KUBIK Interface simulation station, KISS) with leads running from the system into a 20 °C laboratory incubator (Percival E30BHO incubator). The ground reference experiment commenced 2 days after the start of the space experiment and the procedure for the space experiment was replicated: medium injection, first image acquisition, 21-day experiment, fixation, second image acquisition, and cold storage at 4 °C. The temperatures of the ECs measured by temperature logger (see above) on two of the ECs were 3.58 and 4.54 °C during shipment to NASA Ames. During the 21 days main experimental phase, the loggers recorded a temperature of 20.62 °C. During post-experiment storage, the temperature was 3.06 °C.
Sample recovery
Liquid and basalt slide removal from the ECs was performed at the NASA Ames Research Center. From the total of 6 mL of total bulk fluid per EC, an aliquot of 3 mL was taken and 65% nitric acid was added to a final concentration 4% to fix ions and minimise attachment and loss to container walls. These samples were cold stored at 4 °C until further analysis.
Fixative injection was successful for all the space ECs. However, fixative injection failed in four of the ground experiment chambers: one B. subtilis chamber, two C. metallidurans chamber and one non-biological control sample. In these cases, 1 mL of NOTOXhisto was added to the liquid samples before the abovementioned procedures.
In all ECs, two culture chambers were observed to have contamination: an ISS non-biological control chamber in microgravity, juxtaposed to a B. subtilis chamber, was contaminated with cells that were morphologically identical to B. subtilis . In the ground control samples, a non-biological control chamber, juxtaposed to a B. subtilis chamber, had a cellular contaminant at low concentration that formed a white pellet on centrifugation that was morphologically dissimilar to B. subtilis . NOTOXhisto fixation prevented successful DNA extraction and identification in both cases. These data points were removed from the calculations.
All samples were shipped back to the University of Edinburgh in cold storage by Altech Space (Torino, Italy).
ICP-MS analysis of samples
Upon return to Edinburgh, UK, the 3 mL of acid-fixed sample was prepared in the following way: each sample was sequentially (in three batches) spun down in a 1.5 mL tube at 10,000 × g (IEC MicroCL 17 centrifuge, Thermo Scientific) for ten minutes to pellet cells and cell debris. The supernatant was collected into a 15 mL tube and analysed by ICP-MS (inductively coupled plasma mass spectrometry). Acquired liquids were used to determine the bulk fluid REE concentrations. Cell debris pellets were washed two times in ddH 2 O and this discarded liquid was pooled. Nitric acid was added to the pooled fluid to a final concentration of 4%, and the samples were analysed by ICP-MS. This liquid was used to determine the REE concentrations that were washed off the cell matter. The pellet was transferred to an acid-washed glass serum bottle pre-baked at 450 °C in an oven (Carbolite Type 301, UK) for 4 h to remove organic molecules. The vial with the pellet was heated at 450 °C for a further 4 h to volatilise carbon and leave residual ions. After cooling, 1.5 mL of ddH 2 O were added with nitric acid to a final concentration of 4% and samples were analysed by ICP-MS. This liquid was used to determine the REE concentrations associated with the cell material.
ICP-MS analysis was carried out as described below on the R2A 50%, NOTOXhisto and ddH 2 O. It was not possible to examine the separated cryoprotectant for C. metallidurans . However, as a significance enhancement in the biological experiments compared to the controls for this organism was not observed, we infer that the protectant did not add additional REEs.
All samples were analysed by ICP-MS using an Agilent 7500ce (with octopole reaction system), employing an RF (radio-frequency) forward power of 1540 W and reflected power of 1 W, with argon gas flows of 0.81 L/min and 0.20 L/min for carrier and makeup flows, respectively. Sample solutions were taken up into the micro mist nebuliser by peristaltic pump at a rate of approximately 1.2 mL/min. Skimmer and sample cones were made of nickel.
The instrument was operated in spectrum multi-tune acquisition mode and three replicate runs per sample were employed. The isotopes: 139 La, 140 Ce, 141 Pr, 146 Nd, 147 Sm. 153 Eu, 157 Gd, 159 Tb. 163 Dy, 165 Ho, 166 Er, 169 Tm, 172 Yb, 175 Lu were analysed in ‘no gas’ mode with each mass analysed in fully quantification mode and three points per unit mass. The REE Pm (promethium) was not measured as the element is radioactive and no standard was available.
To calibrate the instrument, multi-element calibration standards containing each element were prepared using an REE multi-element standard (Multi-Element Calibration Standard-1, Agilent Technologies, USA) plus a Uranium single-element 1000 mg L −1 standard (SPE Science, Canada) diluted with 2% v/v HNO 3 (Aristar grade, VWR International, United Kingdom). A NIST standard reference material, SRM1640a, was employed as a reference standard for some of the elements. The limits of detection for the REEs were split broadly into two groups: La, Ce, Pr, Tb, Ho, Tm, Lu: 0.0025-0.005 ppb. Nd, Sm, Eu, Gd, Dy, Er, Yb: 0.001-0.005 ppb.
Raw ICP-MS data (determined in μg/L) was converted to obtain the absolute quantity of a given element in the culture chamber, taking into account dilution factors applied during ICP-MS analysis.
To determine REE concentrations in the basalt slide, between 25 and 50 mg of homogenised sample was added to Savillex Teflon vessels. Rock standards (basalt standards BIR-1, BE-N, BCR-2, BHVO-1) were prepared in the same way. Two blanks were included ( i.e ., sample without basalt). Three millilitres of double distilled HNO 3 , 2 mL HCl and 0.5 mL HF was added to each of the vessels. HF was added after the other acids to prevent disassociation, formation and precipitation of aluminium fluorides. Samples were placed on a hot plate for digestion overnight (temperature of 100–120 °C) and checked for complete digestion. Samples were evaporated on the hot plate. Five millilitres of 1 M HNO 3 was added to each vessel. Lids were added and the samples returned to the hot plate for a second digestion step. Samples were further diluted with 2–5% HNO 3 for ICP-MS analysis.
Analysis was carried out on a high resolution, sector field, ICP-MS (Nu AttoM). The ICP-MS measurements for REEs were performed in low resolution (300), in Deflector jump mode with a dwell time of 1 ms and 3 cycles of 500 sweeps. Data were reported in micrograms of REE per gram of basalt.
pH of flight experiment and ground pH experiment
A small aliquot (≈0.3 mL) of liquid from the chambers was used to measure the pH of the solutions at the end of the experiment after fixative addition. The pH was measured using a calibrated Mettler Toledo Semi-Micro-L pH metre. Final values for cell growth in the experiment are reported previously 31 and the values are shown in Supplementary Table 9 .
During the space experiment, only final pH values were obtained. Thus, an experiment was carried out on the ground to investigate the pH changes that might have occurred during the course of the experiment (limited to a 1 × g condition) and the influence of the basalt rock in any observed pH changes.
Sterile basalt slides, as used in the flight experiment, were prepared in 5 mL of 50% R2A in six-well plates (Corning, UK) and the wells were inoculated with one of the three microorganisms used in this study. Control experiments were conducted without organisms, using fresh 50% R2A only, with or without the basalt slide. The experiment was performed at 20 °C for 21 days. After this period, 1 mL of NOTOXhisto was added to each well, and stored at 4 °C for a further week. During the course of the experiment, pH values were measured at fixed intervals (day 0, 1, 4, 7, 14, 21). On the 21st day of the experiment, pH was measured twice, before and after the fixative addition.
Statistical analysis and software
Analysis of the leaching data was performed at several levels of granularity using SPSS Statistics (IBM, version 26). Two and one-way ANOVAs were used to assess significant differences between gravity conditions, organisms, ground and space samples, and between controls, in combinations described in the results. In these analyses, data across all REEs was used (the tests did not discriminate REEs). Data were log 10 transformed and tests for normality of data and equal variances (Levene’s tests) were carried out. Tukey tests were performed where appropriate to examine pairwise comparisons.
To investigate differences between gravity conditions and organisms or controls for specific REEs, a two-sample independent two-tailed Student’s t test was used between pairs of conditions for specific REEs, accepting that the small sample sizes make these tests less reliable than the aggregate ANOVA analyses.
RStudio 1.2.5033 was used to analyse and visualise the ground pH experiment. Microsoft Excel (2016) was used to collect data and Microsoft Word (2016) was used to prepare the manuscript and associated text files.
Data availability
The presented data in the paper are available on the Edinburgh Datashare repository at https://doi.org/10.7488/ds/2908 and from the corresponding author.
Druschel, G. K. & Kappler, A. Geomicrobiology and microbial geochemistry. Elements 11 , 389–394 (2015).
Article Google Scholar
Taunton, A. E., Welch, S. A. & Banfield, J. E. Microbial controls on phosphate and lanthanide distributions during granite weathering and soil formation. Chem. Geol. 169 , 371–382 (2000).
Article ADS CAS Google Scholar
Schulz, S. et al. The role of microorganisms at different stages of ecosystem development for soil formation. Biogeosci . 10 , 3983–3996 (2013).
Article ADS Google Scholar
Kalev, S. D. & Toor, G. S. in Green Chemistry: An Inclusive Approach (eds Torok, B. & Dransfield, T.) 339–357 (Elsevier, 2018).
Rawlings, D. E. & Silver, S. Mining with microbes. Nat. Biotechnol. 13 , 773–778 (1995).
Article CAS Google Scholar
Johnson, D. B. Biomining: biotechnologies for extracting and recovering metals from ores and waste materials. Curr. Opin. Biotechnol. 30 , 24–31 (2014).
Article CAS PubMed Google Scholar
Das, T., Ayyappan, S. & Chaudhury, G. R. Factors affecting bioleaching kinetics of sulfide ores using acidophilic micro-organisms. Biometals 12 , 1–10 (1999).
Hong, Y. & Valix, M. Bioleaching of electronic waste using acidophilic sulfur oxidising bacteria. J. Clean. Prod. 65 , 564–472 (2014).
Hilson, G. & Monhemius, A. J. Alternatives to cyanide in the gold mining industry: what prospects for the future? J. Clean. Prod. 14 , 1158–1167 (2006).
Gu, T., Rastegar, S. O., Mousavi, S. M., Li, M. & Zhou, M. Advances in bioleaching for recovery of metals and bioremediation of fuel ash and sewage sludge. Biores. Technol. 261 , 428–440 (2018).
Reed, D. W., Fujita, Y., Daubaras, D. L., Jiao, Y. & Thompson, V. S. Bioleaching of rare earth elements from waste phosphors and cracking catalysts. Hydrometallurgy 166 , 34–40 (2016).
Bosecker, K. Bioleaching: metal solubilization by microorganisms. FEMS Microbiol. Rev. 20 , 591–604 (1997).
Rezza, I., Salinas, E., Elorza, M., Sanz de Tosetti, M. & Donati, E. Mechanisms involved in bioleaching of an aluminosilicate by heterotrophic microorganisms. Process Biochem. 36 , 495–500 (2001).
Schippers, A. et al. Biomining: metal recovery from ores with microorganisms. Adv. Biochem. Eng. Biotechnol. 141 , 1–47 (2014).
CAS PubMed Google Scholar
Sklodowska, A. & Matlakowska, R. in Microbial Processing of Metal Sulfides (eds Donati, E. R. & Sand, W.) 121–129 (Springer, 2007).
Massari, S. & Ruberti, M. Rare earth elements as critical raw materials: focus on international markets and future strategies. Res. Policy 38 , 36–43 (2013).
Keltjens, J. T., Pol, A., Reimann, J. & Op den Camp, H. J. M. PQQ-dependent methanol dehydrogenases: rare-earth elements make a difference. Appl. Microbiol. Biotechnol. 98 , 6163–6183 (2014).
Huang, J. et al. Rare earth element alcohol dehydrogenases widely occur among globally distributed, numerically abundant and environmentally important microbes. ISME J. 13 , 2005–2017 (2019).
Article PubMed PubMed Central Google Scholar
Pol, A. et al. Rare earth metals are essential for methanotrophic life in volcanic mudpots. Environ. Microbiol. 16 , 255–264 (2014).
Barmettler, F., Castelberg, C., Fabbri, C. & Brandl, H. Microbial mobilization of rare earth elements (REE) from mineral solids—a mini review. AIMS Microbiol. 2 , 190–204 (2016).
Chen, B. et al. An experimental study on the effects of microbes on the migration and accumulation of REE in the weathering crust of granite. Chin. J. Geochem. 19 , art. 280 (2000).
Cockell, C. S. Geomicrobiology beyond Earth—Microbe-mineral interactions in space exploration and settlement. Trends Microbiol. 18 , 308–314 (2010).
Cockell, C. S. Synthetic geomicrobiology: engineering microbe-mineral interactions for space exploration and settlement. Int. J. Astrobiol. 10 , 315–324 (2011).
Montague, M. et al. The role of synthetic biology for In Situ Resource Utilization (ISRU). Astrobiology 12 , 1135–1142 (2012).
Article ADS PubMed Google Scholar
Liu, Y. D. et al. Control of Lunar and Martian dust—experimental insights from artificial and natural cyanobacterial and algal crusts in the desert of Inner Mongolia, China. Astrobiology 8 , 75–86 (2008).
Article ADS CAS PubMed Google Scholar
Mautner, M. N. Biological potential of extraterrestrial materials—1. Nutrients in carbonaceous meteorites, and effects on biological growth. Planet. Space Sci. 45 , 653–661 (1997).
Rothschild, L. J. Synthetic biology meets bioprinting: enabling technologies for humans on Mars (and Earth). Biochem. Soc. Trans. 44 , 1158–1164 (2016).
Article CAS PubMed PubMed Central Google Scholar
Horneck, G., Klaus, D. M. & Mancinelli, R. L. Space microbiology. Microbiol. Mol. Biol. Rev. 74 , 121–156 (2010).
Moissl-Eichinger, C., Cockell, C. S. & Rettberg, P. Venturing into new realms? Microorganisms in space. FEMS Microbiol. Rev. 40 , 722–737 (2016).
Huang, B., Li, D.-G., Huang, Y. & Liu, C.-T. Effects of spaceflight and simulated microgravity on microbial growth and secondary metabolism. Mil. Med. Res. 5 , 18 (2018).
PubMed PubMed Central Google Scholar
Santomartino, R. et al. No effect of microgravity and simulated Mars gravity on final bacterial cell concentrations on the International Space Station: applications to space bioproduction. Fronti. Micriobiol . 11 , 579156 (2020).
Gasset, G. et al. Growth and division of Escherichia coli under microgravity conditions. Res. Microbiol. 145 , 111–120 (1994).
Kacena, M. A. et al. Bacterial growth in space flight: logistic growth curve parameters for Escherichia coli and Bacillus subtilis . Appl. Microbiol. Biotechnol. 51 , 229–234 (1999).
Leys, N. M. E. J., Hendrickx, L., De Boever, P., Baatout, S. & Mergeay, M. Space flight effects on bacterial physiology. J. Biol. Regul. Homeo. Agents 18 , 193–199 (2004).
CAS Google Scholar
Crabbe, A. et al. Spaceflight enhances cell aggregation and random budding in Candida albicans . PLoS ONE 8 , e80677 (2013).
Article ADS CAS PubMed PubMed Central Google Scholar
Ruzicka, A., Snyder, G. A. & Taylor, L. A. Comparative geochemistry of basalts from the Moon, Earth, HED asteroid, and Mars: implications for the origin of the Moon. Geochim. Cosmochim. Acta 65 , 979–997 (2001).
McMahon, S., Parnell, J., Ponicka, J., Hole, M. & Boyce, A. The habitability of vesicles. in martian basalt. Astron. Geophys. 54 , 1.17–1.21 (2013).
McSween, H. Y., Taylor, G. J. & Wyatt, M. B. Elemental composition of the martian crust. Science 324 , 736–739 (2009).
McLean, R. J. C., Cassanto, J. M., Barnes, M. B. & Koo, J. H. Bacterial biofilm formation under microgravity conditions. FEMS Microbiol. Lett. 195 , 115–119 (2001).
Kim, W. et al. Spaceflight promotes biofilm formation by Pseudomonas aeruginosa . PLoS ONE 8 , e62437 (2013).
Pogliani, C. & Donati, E. The role of exopolymers in the bioleaching of a non-ferrous metal sulphide. J. Indus. Microbiol. Biotechnol. 22 , 88–92 (1999).
Welch, S. A., Barker, W. W. & Banfield, J. F. Microbial extracellular polysaccharides and plagioclase dissolution. Geochim. Cosmochim. Acta 63 , 1405–1419 (1999).
Takahashi, Y., Hirata, T., Shimizu, H., Ozaki, T. & Fortin, D. A rare earth element signature of bacteria in natural waters? Chem. Geol. 244 , 569–583 (2007).
White, A. F. et al. The effect of temperature on experimental and natural chemical weathering rates of granitoid rocks. Geochim. Cosmochim. Acta 63 , 3277–3291 (1999).
Rozas, E. E. et al. Bioleaching of electronic waste using bacteria isolated from the marine sponge Hymeniacidon heliophila (Porifera). J. Hazard. Mater. 329 , 120–130 (2017).
Giese, E. C., Carpen, H. L., Bertolino, L. C. & Schneider, C. L. Characterization and bioleaching of nickel laterite ore using Bacillus subtilis strain. Biotechnol. Prog. 35 , e2860 (2019).
Takahashi, Y., Châtellier, X., Hattori, K. H., Kato, K. & Fortin, D. Adsorption of rare earth elements onto bacterial cell walls and its implication for REE sorption onto natural microbial mats. Chem. Geol. 219 , 53–67 (2005).
Kucuker, M. A., Xu, X. & Kuchta, K. in Cascade Use in Technologies 2018 (eds Pehlken, A., Kalverkamp, M. & Wittstock, R.) 45–50 (Springer, 2019).
Byloos, B. et al. The impact of space flight on survival and interaction of Cupriavidus metallidurans CH34 with basalt, a volcanic Moon analog rock. Front. Microbiol. 8 , 671 (2017).
Takahashi, Y. et al. A new method for the determination of CeIII/CeIV ratios in geological materials; application for weathering, sedimentary, and diagenetic processes. Earth Planet. Sci. Lett. 182 , 201–207 (2000).
Xhang, L. et al. Bioleaching of rare earth elements from bastnaesite-bearing rock by actinobacteria. Chem. Geol. 483 , 544–557 (2018).
Rasoulnia, P., Barthen, R. & Lakaniemi, A.-M. A critical review of bioleaching of rare earth elements: the mechanisms and effect of process parameters. Crit. Rev. Env. Sci. Technol . https://doi.org/10.1080/10643389.2020.1727718 (2020).
Willscher, S. & Bosecker, K. Studies on the leaching behaviour of heterotrophic microorganisms isolated from an alkaline slag dump. Hydromet 71 , 257–264 (2003).
Lapanje, A., Wimmersberger, C., Furrer, G., Brunner, I. & Frey, B. Pattern of elemental release during the granite dissolution can be changed by aerobic heterotrophic bacterial strains isolated from Damma Glacier (Central Alps) deglaciated granite sand. Soil Microbiol. 63 , 865–882 (2011).
Google Scholar
McLeod, C. L. & Krekeler, M. P. S. Sources of extraterrestrial rare earth alements: to the Moon and beyond. Resources 6 , 40 (2017).
Wieczorek, M. A. & Phillips, R. J. The “Procellarum KREEP Terrane”: Implications for mare volcanism and lunar evolution. J. Geophys. Res. 105 , 20417–20430 (2000).
Mautner, M. N., Conner, A. J., Killham, K. & Deamer, D. W. Biological potential of extraterrestrial materials. Icarus 129 , 245–253 (1997).
González-Toril, E. et al. Iron meteorites can support the growth of acidophilic chemolithoautotrophic microorganisms. Astrobiology 5 , 406–414 (2005).
Gronstal, A. L. et al. Laboratory experiments on the weathering of iron meteorites and carbonaceous chondrites by iron-oxidising bacteria. Meteorit. Planet. Sci. 44 , 233–248 (2009).
Milojevic, T. et al. Exploring the microbial biotransformation of extraterrestrial material on nanometer scale. Sci. Rep. 9 , 18028 (2019).
Loudon, C.-M. et al. BioRock: new experiments and hardware to investigate microbe–mineral interactions in space. Int. J. Astrobiol. 17 , 303–313 (2018).
Reddy, G. S. N. & Garcia-Pichel, F. Sphingomonas mucosissima sp. nov. and Sphingomonas desiccabilis sp. nov., from biological soil crusts in the Colorado Plateau, US. Int. J. Syst. Evol. Microbiol 57 , 1028–1034 (2007).
Song, W., Ogawab, N., Oguchic, C. T., Hattad, T. & Matsukuraa, Y. Effect of Bacillus subtilis on granite weathering: a laboratory experiment. CATENA 70 , 275–281 (2007).
Diels, L. & Mergeay, M. DNA probe-mediated detection of resistant bacteria from soils highly polluted by heavy metals. Appl. Environ. Microbiol 56 , 1485–1491 (1990).
Brim, H. et al. Amplified rDNA restriction analysis and further genotypic characterisation of metal-resistant soil bacteria and related facultative hydrogenotrophs. Syst. Appl. Microbiol. 22 , 258–268 (1999).
Goris, J. et al. Classification of metal-resistant bacteria from industrial biotopes as Ralstonia campinensis sp. nov., Ralstonia metallidurans sp. nov. and Ralstonia basilensis Steinle et al. 1998 emend. Int. J. Syst. Evol. Microbiol. 51 , 1773–1782 (2001).
Sahl, J. W. et al. Subsurface microbial diversity in deep-granitic-fracture water in Colorado. Appl. Environ. Microbiol. 74 , 143–152 (2008).
Kelly, L. et al. Bacterial diversity of terrestrial crystalline volcanic rocks, Iceland. Microb. Ecol. 62 , 69–79 (2011).
Mijnendonckx, K. et al. Characterization of the survival ability of Cupriavidus metallidurans and Ralstonia pickettii from space-related environments. Microb. Ecol. 65 , 347–360 (2013).
Leys, N. et al. The response of Cupriavidus metallidurans CH34 to spaceflight in the International Space Station. Antonie Van. Leeuwenhoek 96 , 227–245 (2009).
Reasoner, D. J. & Geldreich, E. E. A new medium for the enumeration and subculture of bacteria from potable water. Appl. Environ. Microbiol 49 , 1–7 (1985).
Fuchs, F. M., Driks, A., Setlow, P. & Moeller, R. An improved protocol for harvesting Bacillus subtilis colony biofilms. J. Microbiol. Methods 134 , 7–13 (2017).
Download references
Acknowledgements
C.S.C., R.S. and the preparation of the experiment and post-flight analysis were funded by UK Science and Technology Facilities Council under grant ST/R000875/1. AW was supported by a Principal’s Career Development PhD Scholarship. R.M., F.M.F. and P.R. were supported by the DLR grant “DLR-FuE-Projekt ISS LIFE, Programm RF-FuW, Teilprogramm 475”. F.M.F. was also supported by the Helmholtz Space Life Sciences Research School at DLR. R.V.H. and N.L. received financial support for this study from Belspo and ESA through the PRODEX EGEM/Biorock project contract (PEA 4000011082). We thank Laetitia Pichevin for ICP-MS analysis of the basalt substrate. We thank the European Space Agency (ESA) for offering the flight opportunity. A special thanks to the dedicated ESA/ESTEC teams, Kayser Italia s.r.l., and the USOC BIOTESC for the development, integration and operation effort. We are thankful to the UK Space Agency (UKSA) for the national support to the project, NASA Kennedy for their support in the experiment integration prior to the SpaceX Falcon 9 CSR-18 rocket launch, particularly Kamber Scott and Anne Currin, and NASA Ames for hosting the ground control experiment. We thank SpaceX and Elon Musk for launching our mining experiment into space.
Author information
These authors contributed equally: Charles S. Cockell, Rosa Santomartino.
Authors and Affiliations
UK Centre for Astrobiology, School of Physics and Astronomy, University of Edinburgh, Edinburgh, UK
Charles S. Cockell, Rosa Santomartino, Annemiek C. Waajen, Natasha Nicholson & Claire-Marie Loudon
Department of Bioscience–Microbiology, Ny Munkegade 116, Building 1540, 129, 8000, Aarhus C, Denmark
Kai Finster
School of Chemistry, University of Edinburgh, Edinburgh, UK
Lorna J. Eades
Radiation Biology Department, German Aerospace Center (DLR), Institute of Aerospace Medicine, Linder Hoehe, Köln, Germany
Ralf Moeller, Petra Rettberg & Felix M. Fuchs
Institute of Electrical Engineering and Plasma Technology, Faculty of Electrical Engineering and Information Sciences, Ruhr University Bochum, Bochum, Germany
Felix M. Fuchs
Microbiology Unit, Belgian Nuclear Research Centre, SCK CEN, Mol, Belgium
Rob Van Houdt, Natalie Leys & Ilse Coninx
ESTEC, Keplerlaan 1, 2201 AZ, Noordwijk, Netherlands
Jason Hatton, Luca Parmitano, Jutta Krause, Andrea Koehler, Nicol Caplin, Lobke Zuijderduijn & René Demets
Kayser Italia S.r.l., Via di Popogna, 501, 57128, Livorno, Italy
Alessandro Mariani, Stefano S. Pellari, Fabrizio Carubia, Giacomo Luciani, Michele Balsamo & Valfredo Zolesi
BIOTESC, Hochschule Luzern Technik & Architektur, Lucerne School of Engineering and Architecture, Obermattweg 9, 6052, Hergiswil, Switzerland
Jeannine Doswald-Winkler, Magdalena Herová & Bernd Rattenbacher
Exobiology Branch, NASA Ames Research Center, Moffett Field, CA, USA
Jennifer Wadsworth & R. Craig Everroad
You can also search for this author in PubMed Google Scholar
Contributions
C.S.C. conceived the BioRock experiment in the framework of the ESA topical team Geomicrobiology for Space Settlement and Exploration (GESSE). C.S.C., R.S. and K.F. designed the experiments for this paper. N.N. and C.M.L. carried out ground experiments and studies in preparation for flight. C.S.C., R.S. and A.C.W. integrated the hardware for spaceflight and ground controls. C.S.C., R.S. and L.J.E. produced the experimental data. C.S.C. and R.S. performed the data analyses. R.M., P.R., F.F. and R.V.H., N.L., I.C. provided B. subtilis and C. metallidurans samples, respectively. L.P. performed the procedures on-board the ISS. R.D., J.H., J.K., A.K., N.C. and L.Z. supervised the technical organisation and implementation of the experiment at ESA. J.D.W., M.H. and B.R. supervised the flight procedures. A.M., S.P., F.C., G.L., M.B. and V.Z. designed and fabricated the hardware. R.C.E. and J.W. hosted the ground control experiment. C.S.C. wrote the manuscript. All authors discussed the results and commented on the manuscript. C.S.C. and R.S. contributed equally to the work.
Corresponding author
Correspondence to Charles S. Cockell .
Ethics declarations
Competing interests.
Authors V.Z., M.B., A.M., S.S.P., F.C. and G.L. were employed by the company Kayser Italia S.r.l. The remaining authors declare no competing interests.
Additional information
Peer review information Nature Communications thanks Anna Kaksonen, Robert McLean, and Elizabeth Watkin for their contributions to the peer review of this work. Peer review reports are available.
Publisher’s note Springer Nature remains neutral with regard to jurisdictional claims in published maps and institutional affiliations.
Supplementary information
Supplementary information, peer review file, rights and permissions.
Open Access This article is licensed under a Creative Commons Attribution 4.0 International License, which permits use, sharing, adaptation, distribution and reproduction in any medium or format, as long as you give appropriate credit to the original author(s) and the source, provide a link to the Creative Commons license, and indicate if changes were made. The images or other third party material in this article are included in the article’s Creative Commons license, unless indicated otherwise in a credit line to the material. If material is not included in the article’s Creative Commons license and your intended use is not permitted by statutory regulation or exceeds the permitted use, you will need to obtain permission directly from the copyright holder. To view a copy of this license, visit http://creativecommons.org/licenses/by/4.0/ .
Reprints and permissions
About this article
Cite this article.
Cockell, C.S., Santomartino, R., Finster, K. et al. Space station biomining experiment demonstrates rare earth element extraction in microgravity and Mars gravity. Nat Commun 11 , 5523 (2020). https://doi.org/10.1038/s41467-020-19276-w
Download citation
Received : 03 July 2020
Accepted : 07 October 2020
Published : 10 November 2020
DOI : https://doi.org/10.1038/s41467-020-19276-w
Share this article
Anyone you share the following link with will be able to read this content:
Sorry, a shareable link is not currently available for this article.
Provided by the Springer Nature SharedIt content-sharing initiative
This article is cited by
Organic carbon source controlled microbial olivine dissolution in small-scale flow-through bioreactors, for co2 removal.
- Thomas D. W. Corbett
- Marcus Westholm
- Anna Neubeck
npj Materials Degradation (2024)
Synthetic microbiology in sustainability applications
- Ethan M. Jones
- John P. Marken
- Pamela A. Silver
Nature Reviews Microbiology (2024)
Siderite and vivianite as energy sources for the extreme acidophilic bacterium Acidithiobacillus ferrooxidans in the context of mars habitability
- Gabriel Gonçalves Silva
- Roberta Almeida Vincenzi
- Fabio Rodrigues
Scientific Reports (2024)
Space bioprocess engineering as a potential catalyst for sustainability
- Gretchen Vengerova
- Isaac Lipsky
- Aaron J. Berliner
Nature Sustainability (2024)
Observation of a promethium complex in solution
- Darren M. Driscoll
- Frankie D. White
- Alexander S. Ivanov
Nature (2024)
By submitting a comment you agree to abide by our Terms and Community Guidelines . If you find something abusive or that does not comply with our terms or guidelines please flag it as inappropriate.
Quick links
- Explore articles by subject
- Guide to authors
- Editorial policies
Sign up for the Nature Briefing: Translational Research newsletter — top stories in biotechnology, drug discovery and pharma.

Academia.edu no longer supports Internet Explorer.
To browse Academia.edu and the wider internet faster and more securely, please take a few seconds to upgrade your browser .
Enter the email address you signed up with and we'll email you a reset link.
- We're Hiring!
- Help Center
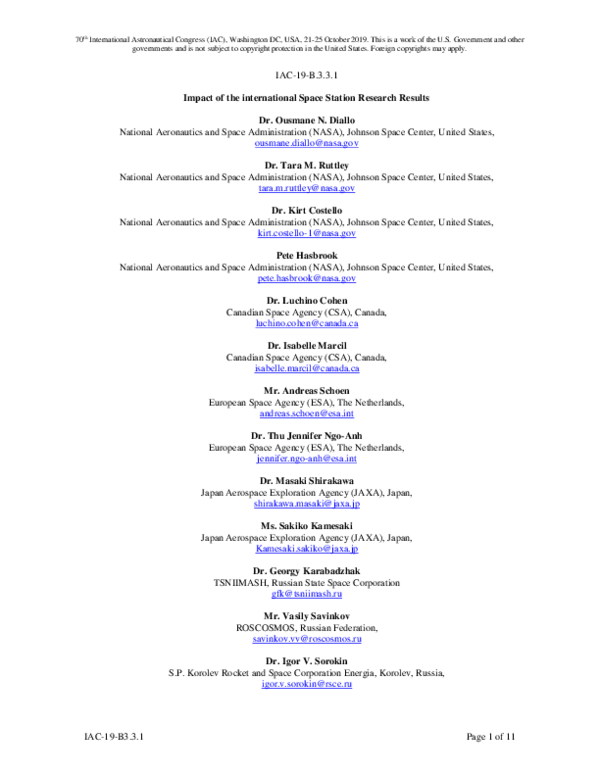
Impact of the International Space Station Research Results

2019, International Astronautical Congress (IAC)
Related Papers
Acta Astronautica
John-David Bartoe , John Uri
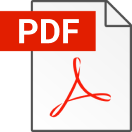
Susan Mayo , Julie A Robinson , Sayaka Umemura , Tracy Parr , Salvatore Pignataro , Martin Zell , Nicole Buckley
44th AIAA Aerospace Sciences Meeting and Exhibit
Julie A Robinson
47th AIAA Aerospace Sciences Meeting including The New Horizons Forum and Aerospace Exposition
Cynthia Evans
The International Space Station (ISS) is a unique scientific platform that enables researchers from all over the world to put their talents to work on innovative experiments that could not be done anywhere else. Although each space station partner has distinct agency goals for station research, each partner shares a unified goal to extend the resulting knowledge for the betterment of humanity. We may not know yet what will be the most important discovery gained from the space station, but we already have some amazing breakthroughs. In the areas of human health, innovative technology, education and observations of Earth from space, there are already demonstrated benefits to people back on Earth. Lives have been saved, station-generated images assist with disaster relief, new materials improve products, and education programs inspire future scientists, engineers and space explorers. Some benefits in this updated second edition have expanded in scope. In other cases, new benefits have ...
Tara Ruttley , Julie A Robinson , Cynthia Evans
46th AIAA Aerospace Sciences Meeting and Exhibit
Tara Ruttley
Gary Kitmacher , William Gerstenmaier , Nicholas Mustachio , John-David Bartoe
Nearly six years after the launch of the first International Space Station element, and four years after its initial occupation, the United States and our 6 international partners have made great strides in operating this impressive Earth orbiting research facility. This past year we have done so in the face of the adversity of operating without the benefit of the Space Shuttle. In his January 14, 2004, speech announcing a new vision for America's space program, President Bush affirmed the United States’ commitment to completing construction of the International Space Station by 2010. The President also stated that we would focus our future research aboard the Station on the long-term effects of space travel on human biology. This research will help enable human crews to venture through the vast voids of space for months at a time. In addition, ISS affords a unique opportunity to serve as an engineering test bed for hardware and operations critical to the exploration tasks. NASA looks forward to working with our partners on International Space Station research that will help open up new pathways for future exploration and discovery beyond low Earth orbit. This paper provides an overview of the International Space Station Program focusing on a review of the events of the past year, as well as plans for next year and the future.
Loading Preview
Sorry, preview is currently unavailable. You can download the paper by clicking the button above.
RELATED PAPERS
45th AIAA Aerospace Sciences Meeting and Exhibit
Judy Tate-Brown
Tracy Parr , Julie A Robinson
… Conference, 2005 IEEE
William Gilbreath
49th AIAA Aerospace Sciences Meeting including the New Horizons Forum and Aerospace Exposition
Microgravity Science …
Tabowei Churchill Onuosa
NASA, TP-2006-213721, …
The International Archives of the Photogrammetry, Remote Sensing and Spatial Information Sciences
Valerio Tramutoli
AIAA SPACE 2009 Conference & Exposition
Harley Thronson
2011 Aerospace Conference
Quang-Viet Nguyen
Leopold Summerer
RELATED TOPICS
- We're Hiring!
- Help Center
- Find new research papers in:
- Health Sciences
- Earth Sciences
- Cognitive Science
- Mathematics
- Computer Science
- Academia ©2024

IEEE Account
- Change Username/Password
- Update Address
Purchase Details
- Payment Options
- Order History
- View Purchased Documents
Profile Information
- Communications Preferences
- Profession and Education
- Technical Interests
- US & Canada: +1 800 678 4333
- Worldwide: +1 732 981 0060
- Contact & Support
- About IEEE Xplore
- Accessibility
- Terms of Use
- Nondiscrimination Policy
- Privacy & Opting Out of Cookies
A not-for-profit organization, IEEE is the world's largest technical professional organization dedicated to advancing technology for the benefit of humanity. © Copyright 2024 IEEE - All rights reserved. Use of this web site signifies your agreement to the terms and conditions.
Subscribe to our free Newsletter! →
Home › Science & Technology News › Astronomy News
Space archaeology takes flight in pioneering ISS study
By StudyFinds Staff
Reviewed by Steve Fink
Research led by Justin Walsh, Chapman University
Aug 13, 2024

International Space Station (credit: NASA/Unsplash)
ORANGE, Calif. — Archaeology has officially entered the Space Age. In a groundbreaking study, researchers have conducted the first archaeological investigation of the International Space Station, shedding new light on how humans live and work without gravity.
The project, dubbed the Sampling Quadrangle Assemblages Research Experiment (SQuARE), aimed to understand how astronauts use and adapt spaces on the ISS, often in ways that differ from the station’s designers’ original intentions. This innovative approach to studying life in space could have far-reaching implications for the design of future space habitats and long-duration space missions .
Led by Justin Walsh from Chapman University, the research team analyzed two specific areas on the ISS over a 60-day period in early 2022. By examining the placement and movement of objects in these areas, the archaeologists were able to piece together a picture of how astronauts actually use these spaces in their daily lives.
One of the most striking findings, published in PLoS ONE , was that areas designed for specific purposes often end up being used quite differently in practice. For example, a workstation intended for equipment maintenance became primarily a storage area, with tools and supplies often left untouched for long periods. This highlights the importance of flexible, multi-purpose spaces in future space habitat designs .

The study also revealed the ingenious ways astronauts cope with the challenges of microgravity . In the absence of gravity to keep objects in place, crew members employ various “gravity surrogates” – items like clips, bungee cords, and Velcro patches that help secure objects to walls and surfaces. These makeshift solutions made up a significant portion of the items observed in the study areas, underscoring their critical role in daily life aboard the ISS.
Another intriguing discovery was how astronauts adapt spaces for personal use. In one area near exercise equipment and the station’s bathroom, researchers observed a toiletry kit left out for nearly two months. This improvised personal care station suggests that current space station designs may not adequately account for the crew’s needs for privacy and personal grooming.
These findings have important implications for future space exploration . As humans set their sights on long-term missions to the Moon and Mars, understanding how to design living spaces that truly meet the needs of space travelers becomes crucial. The SQuARE project demonstrates that archaeological methods can provide valuable insights that go beyond what can be learned from interviews or predetermined metrics.
Moreover, this research opens up an entirely new field of study: space archaeology . Just as terrestrial archaeology helps us understand how ancient peoples lived and adapted to their environments, space archaeology can shed light on how humans adapt to the unique challenges of off-world habitats. This could prove invaluable as we continue to push the boundaries of human space exploration.

“The experiment is the first archaeology ever to happen off of the planet Earth. By applying a very traditional method for sampling a site to a completely new kind of archaeological context, we show how the ISS crew uses different areas of the space station in ways that diverge from designs and mission plans. Architects and planners of future space stations can learn valuable lessons from this work,” the researchers write in a media release.
The SQuARE project marks a significant milestone in our understanding of life in space. By bringing archaeological methods to bear on the ISS, researchers have uncovered new insights into how humans adapt to and shape their environment in microgravity. As we look to the future of space exploration, these findings could help inform the design of more effective and livable space habitats, paving the way for humanity’s long-term presence beyond Earth.
Paper Summary
Methodology.
The researchers selected six locations on the ISS and asked astronauts to photograph these areas daily for 60 days. They focused on two of these areas for this initial study: a maintenance workstation and an area near exercise equipment and the bathroom. Using special software, they identified and categorized every visible object in each photo, tracking how items moved or changed over time. This allowed them to build a detailed picture of how these spaces were used day-to-day, much like how terrestrial archaeologists study layers of soil to understand how ancient sites were used over time.
Key Results
The study revealed that the maintenance workstation was used more for storage than actual maintenance work. Many tools remained untouched throughout the study period. In the area near the exercise equipment, personal hygiene items were frequently present, suggesting this space was often used for grooming despite not being designed for that purpose. In both areas, items used to secure objects in microgravity (like Velcro and bungee cords) were abundant, highlighting their importance in daily life on the ISS.
Study Limitations
The study only looked at two areas on the ISS for a limited time period, so it may not represent all aspects of life on the station. The researchers also didn’t have access to detailed crew schedules, which could have provided additional context for the observed activities. Furthermore, the presence of the study itself may have influenced crew behavior in the photographed areas.
Discussion & Takeaways
The researchers concluded that flexibility is key in space habitat design. Areas often end up being used differently than intended, so multi-purpose spaces could be more effective. They also highlighted the need for better personal care facilities and storage solutions in future space habitats. The study demonstrated the value of archaeological methods in understanding life in space, potentially opening up a new field of space archaeology.
Funding & Disclosures
The study was funded by Chapman University’s Office of Research and Sponsored Programs. The International Space Station National Laboratory provided access to the ISS and crew time. The researchers declared no competing interests. The study was conducted with the cooperation of NASA and international space agencies, with Axiom Space serving as the implementation partner for the research.
About StudyFinds Staff
StudyFinds sets out to find new research that speaks to mass audiences — without all the scientific jargon. The stories we publish are digestible, summarized versions of research that are intended to inform the reader as well as stir civil, educated debate. StudyFinds Staff articles are AI assisted, but always thoroughly reviewed and edited by a Study Finds staff member. Read our AI Policy for more information.
Our Editorial Process
StudyFinds publishes digestible, agenda-free, transparent research summaries that are intended to inform the reader as well as stir civil, educated debate. We do not agree nor disagree with any of the studies we post, rather, we encourage our readers to debate the veracity of the findings themselves. All articles published on StudyFinds are vetted by our editors prior to publication and include links back to the source or corresponding journal article, if possible.
Our Editorial Team
Editor-in-Chief
Chris Melore
Sophia Naughton
Associate Editor
Related Content

Sickening sink secrets: The hidden bacterial world in your basin
August 15, 2024

Milky Way galaxy may dodge looming collision with Andromeda

Your plate is your medicine cabinet: How ancient wisdom is transforming modern diets
Leave a reply cancel reply.

©2024 Study Finds. All rights reserved. Privacy Policy • Disclosure Policy • Do Not Sell My Personal Information
New ISS research project will benefit cancer patients on Earth
The NASA partnership is spotlighting cancer research in space.
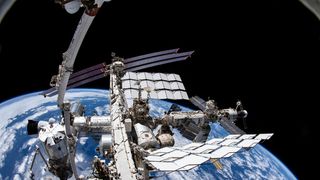
Since 2013, research has been underway on the International Space Station (ISS) National Lab with astronauts conducting experiments in microgravity to ultimately help improve our lives here on Earth .
Diseases like cancer remain one of the top causes of death across the United States and, in a recent release , the National Cancer Institute estimated that this year alone will see more than 2 million cancer cases diagnosed with a death toll of more than 600,000 people. With numbers like that, even medical research in space has been focused on cancer-related treatment.
At the end of July, the ISS National Lab shared in the same release that more than $7 million in total funding through the Cancer Moonshot initiative was awarded to five research teams selected through the ISS National Lab and National Aeronautics and Space Administration (NASA)'s Biological and Physical Sciences (BPS) division . The projects included are Eascra Biotech , Cedars-Sinai Medical Center , University of California, San Diego , Wake Forest Institute for Regenerative Medicine (WFIRM) and University of Texas MD Anderson Cancer Center . Each will use the rotating microgravity lab to further cancer research.
"Over the years, the space station has been a catalyst for biomedical research that has profound impacts on patient care on Earth," Ray Lugo, chief executive officer for the Center for the Advancement of Science in Space and manager of the ISS National Lab, said in the release. "Through this inaugural Igniting Innovation research announcement, the ISS National Lab and NASA focused funding efforts to specifically target cancer through space-based research, and we look forward to working with the selected projects as they push the boundaries of research and innovation to develop more effective therapeutics for those impacted by this devastating disease."
Related: ISS scientists make headway in cancer research: 'We're NASA, we do Moonshots'
Space.com had an exclusive opportunity to speak with the team at Deep Space Biology , a California-based company selected to be part of the MD Anderson Cancer Center project that will specifically focus on researching how T cells are changed in microgravity. The multi-year research will be a collaboration with Axiom Space , BioServe Space Technologies and Mongoose Bio , focusing on discovering new biological advances and enhancing cancer treatments across the globe.
"At Axiom Space, we are proud to support the pioneering work led by Deep Space Biology. This groundbreaking artificial intelligence technology marks a significant leap forward for the space biotech industry,” Matt Ondler, president of Axiom Space , told Space.com in an email. "By leveraging the unique environment of space , we are uncovering new insights and developing innovative treatments that have the potential to revolutionize healthcare on Earth. The future of space biotech is incredibly promising, and we are excited to be part of this transformative journey."
Get the Space.com Newsletter
Breaking space news, the latest updates on rocket launches, skywatching events and more!
Deep Space Biology has developed the first-ever approach to space-based biotech using artificial intelligence. The cutting-edge platform, Yotta, uses AI to pinpoint important disease targets and make comparisons between different T-cell experiments in microgravity as well as on Earth . By using AI algorithms, researchers will be able to have large amounts of biological data analyzed at a much faster pace, which will lead to more timely creation and validation of new medical advances.
— How Space Science Is Helping Fight Against Cancer
— Cancer-Fighting Drug, Algae and Mice Headed to International Space Station on SpaceX Dragon
— The Apollo program continues to inspire 'moonshots' in the 21st century
"Amazing things are possible when great teams work together. Our collaboration with MD Anderson represents a significant step forward in leveraging space-based research to revolutionize cancer and immunology studies," Montana Bilger, co-founder and CEO of Deep Space Biology, told Space.com by email. "By integrating Deep Space Biology's AI technology, we are excited to uncover groundbreaking insights that will propel medical science to new heights. The Yotta platform is designed to revolutionize pharmaceutical research by providing unprecedented insights and accelerating the discovery of therapeutics for complex diseases like cancer."
Space biology research has been underway for more than 20 years and continues to contribute to making life-changing discoveries. This research can be incorporated into the healthcare industry and provide more knowledge on disease treatments and aim to cure the most complex conditions.
Join our Space Forums to keep talking space on the latest missions, night sky and more! And if you have a news tip, correction or comment, let us know at: [email protected].
Meredith is a regional Murrow award-winning Certified Broadcast Meteorologist and science/space correspondent. She most recently was a Freelance Meteorologist for NY 1 in New York City & the 19 First Alert Weather Team in Cleveland. A self-described "Rocket Girl," Meredith's personal and professional work has drawn recognition over the last decade, including the inaugural Valparaiso University Alumni Association First Decade Achievement Award, two special reports in News 12's Climate Special "Saving Our Shores" that won a Regional Edward R. Murrow Award, multiple Fair Media Council Folio & Press Club of Long Island awards for meteorology & reporting, and a Long Island Business News & NYC TV Week "40 Under 40" Award.
Listen live: NASA providing update about troubled Boeing Starliner astronaut mission today
Archaeology on the ISS? Scientists study how astronauts use and store stuff in space
SpaceX to launch 1st space-hardened Nvidia AI GPU on upcoming rideshare mission
Most Popular
- 2 Listen live: NASA providing update about troubled Boeing Starliner astronaut mission today
- 3 Weird, 'watermelon shape' asteroids like Dimorphos and Selam may finally have an explanation
- 4 New ISS images showcase auroras, moon and space station in glorious photos (video)
- 5 Watch a Perseid fireball light up the skies above Macedonia in this striking video
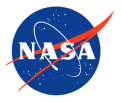
Suggested Searches
- Climate Change
- Expedition 64
- Mars perseverance
- SpaceX Crew-2
International Space Station
- View All Topics A-Z
Humans in Space
Earth & climate, the solar system, the universe, aeronautics, learning resources, news & events.
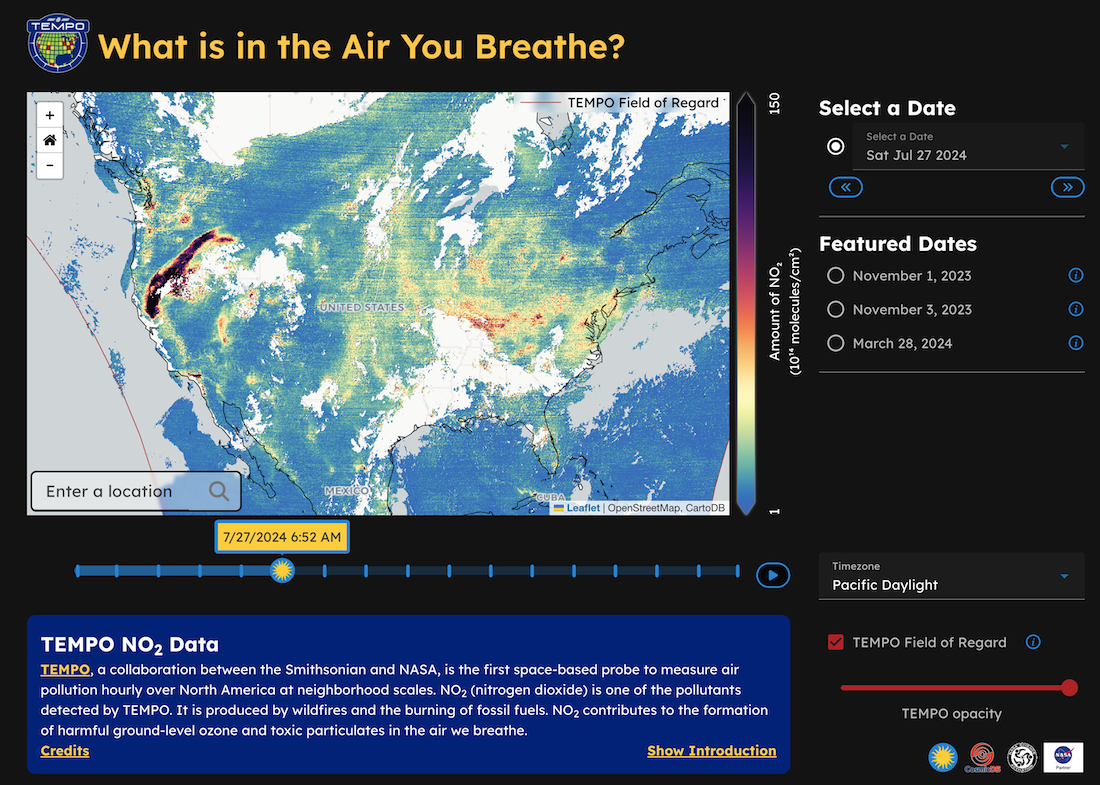
New TEMPO Cosmic Data Story Makes Air Quality Data Publicly Available
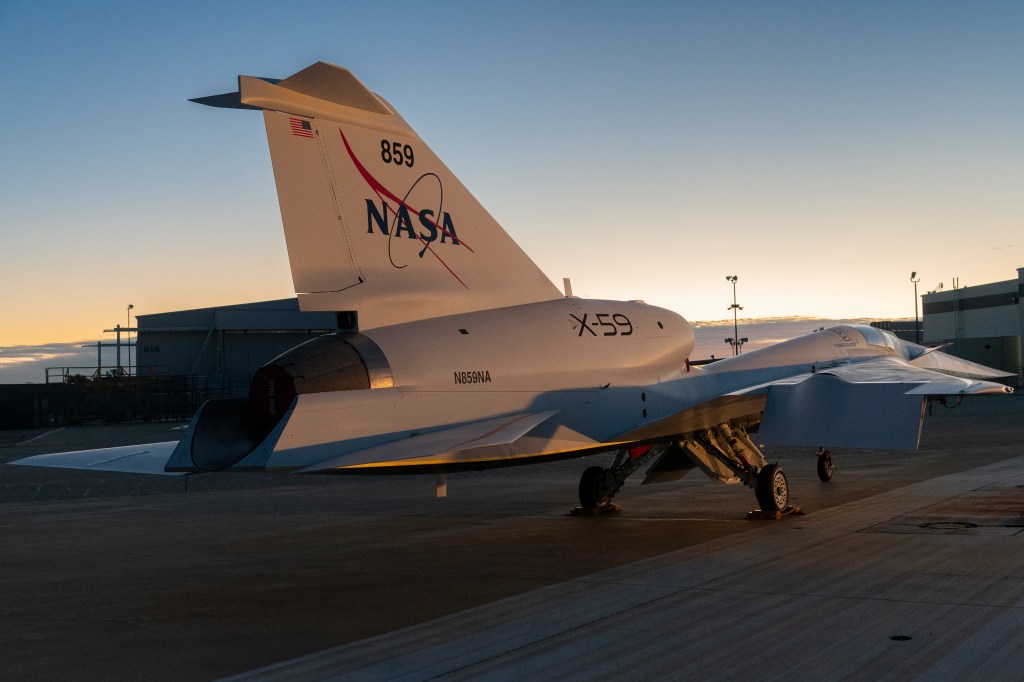
NASA’s X-59 Progresses Through Tests on the Path to Flight

NASA Demonstrates ‘Ultra-Cool’ Quantum Sensor for First Time in Space
- Search All NASA Missions
- A to Z List of Missions
- Upcoming Launches and Landings
- Spaceships and Rockets
- Communicating with Missions
- James Webb Space Telescope
- Hubble Space Telescope
- Why Go to Space
- Commercial Space
- Destinations
- Living in Space
- Explore Earth Science
- Earth, Our Planet
- Earth Science in Action
- Earth Multimedia
- Earth Science Researchers
- Pluto & Dwarf Planets
- Asteroids, Comets & Meteors
- The Kuiper Belt
- The Oort Cloud
- Skywatching
- The Search for Life in the Universe
- Black Holes
- The Big Bang
- Dark Energy & Dark Matter
- Earth Science
- Planetary Science
- Astrophysics & Space Science
- The Sun & Heliophysics
- Biological & Physical Sciences
- Lunar Science
- Citizen Science
- Astromaterials
- Aeronautics Research
- Human Space Travel Research
- Science in the Air
- NASA Aircraft
- Flight Innovation
- Supersonic Flight
- Air Traffic Solutions
- Green Aviation Tech
- Drones & You
- Technology Transfer & Spinoffs
- Space Travel Technology
- Technology Living in Space
- Manufacturing and Materials
- Science Instruments
- For Kids and Students
- For Educators
- For Colleges and Universities
- For Professionals
- Science for Everyone
- Requests for Exhibits, Artifacts, or Speakers
- STEM Engagement at NASA
- NASA's Impacts
- Centers and Facilities
- Directorates
- Organizations
- People of NASA
- Internships
- Our History
- Doing Business with NASA
- Get Involved
NASA en Español
- Aeronáutica
- Ciencias Terrestres
- Sistema Solar
- All NASA News
- Video Series on NASA+
- Newsletters
- Social Media
- Media Resources
- Upcoming Launches & Landings
- Virtual Events
- Sounds and Ringtones
- Interactives
- STEM Multimedia
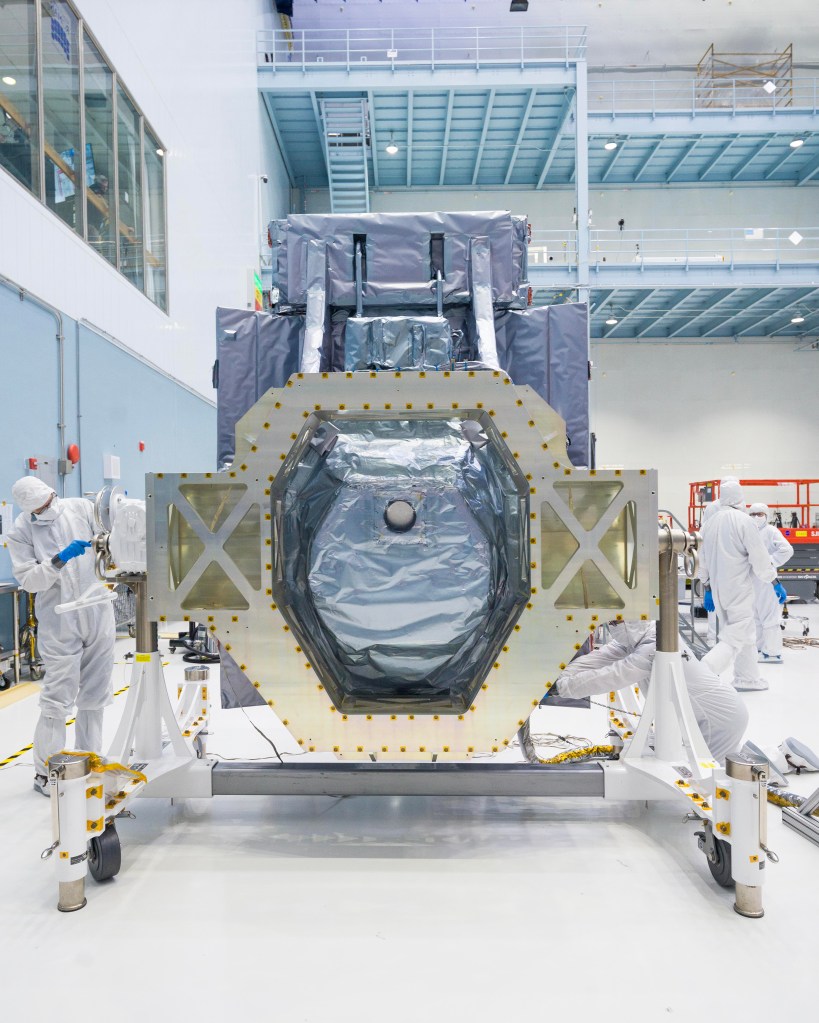
Primary Instrument for Roman Space Telescope Arrives at NASA Goddard
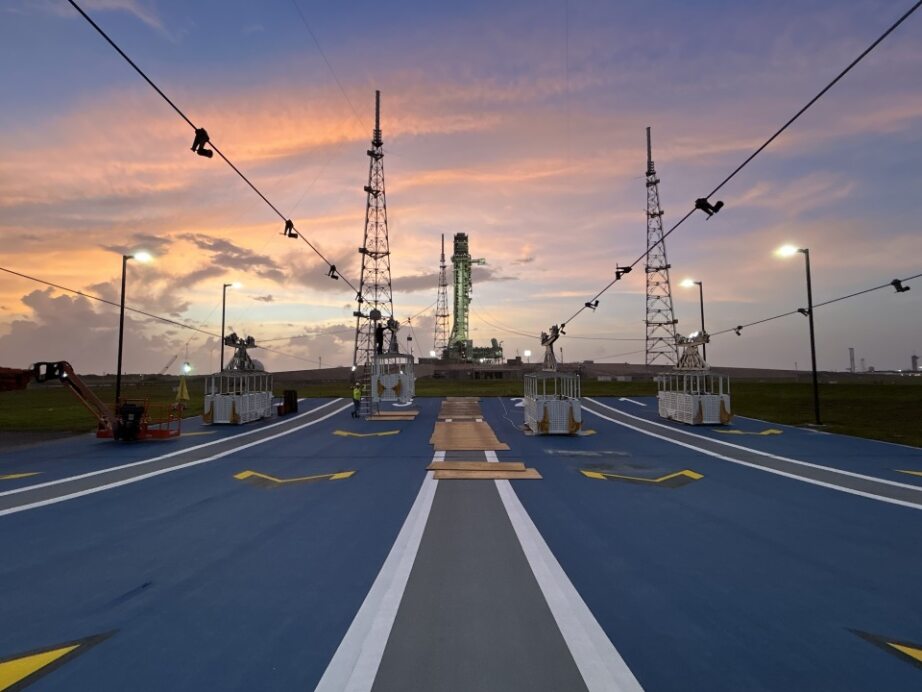
Artemis Emergency Egress System Emphasizes Crew Safety
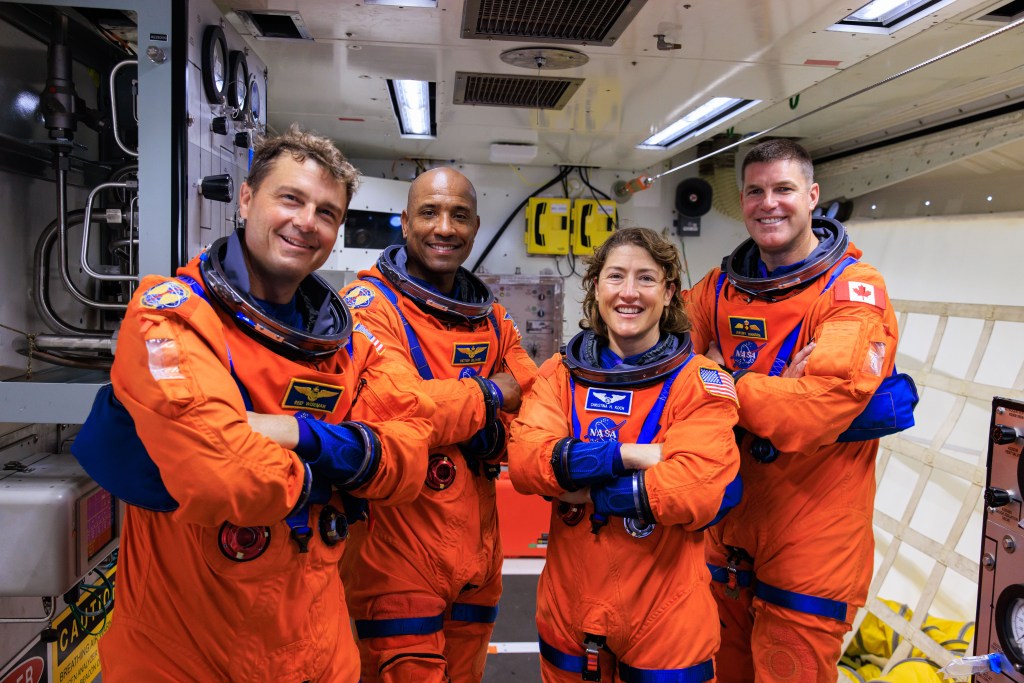
What’s New With the Artemis II Crew
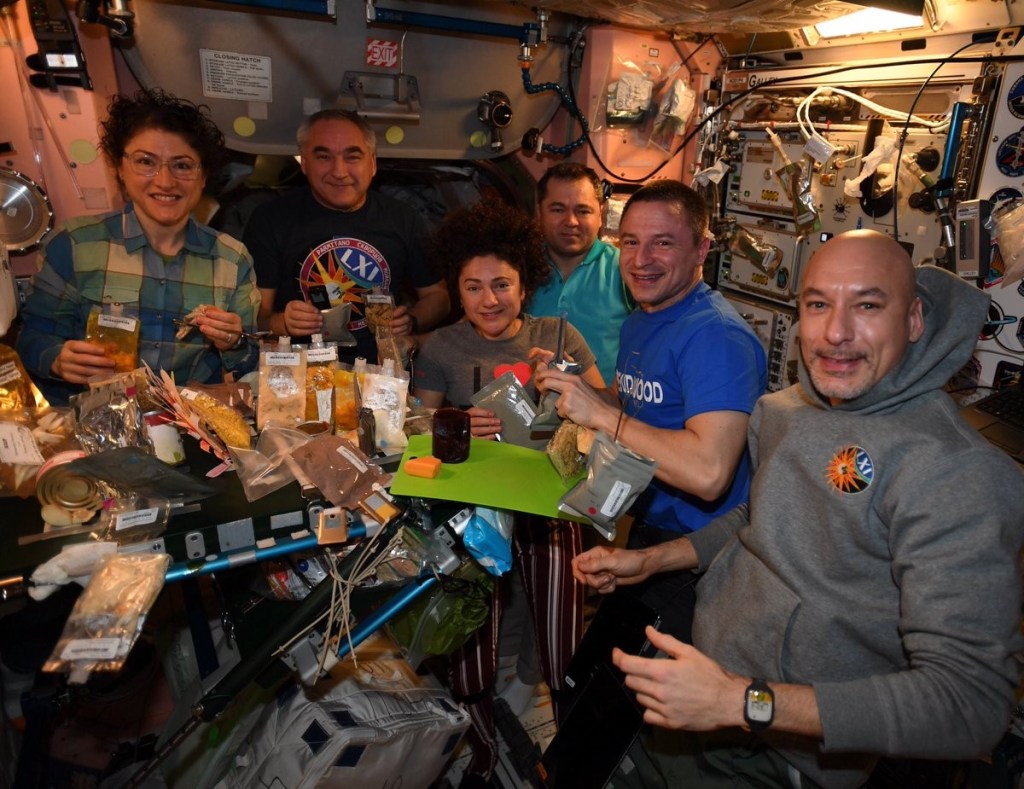
Food in Space
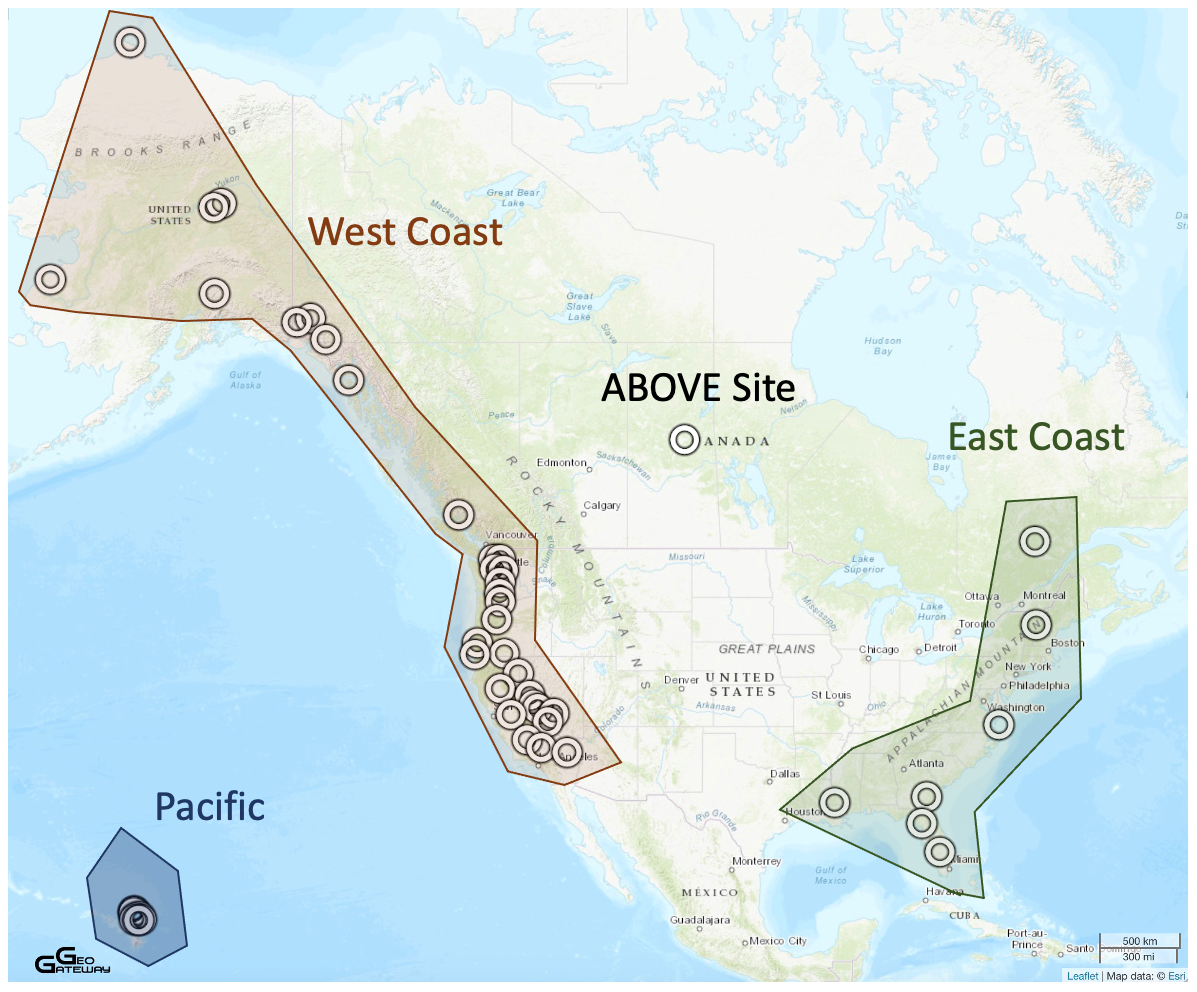
Airborne Surface, Cryosphere, Ecosystem, and Nearshore Topography
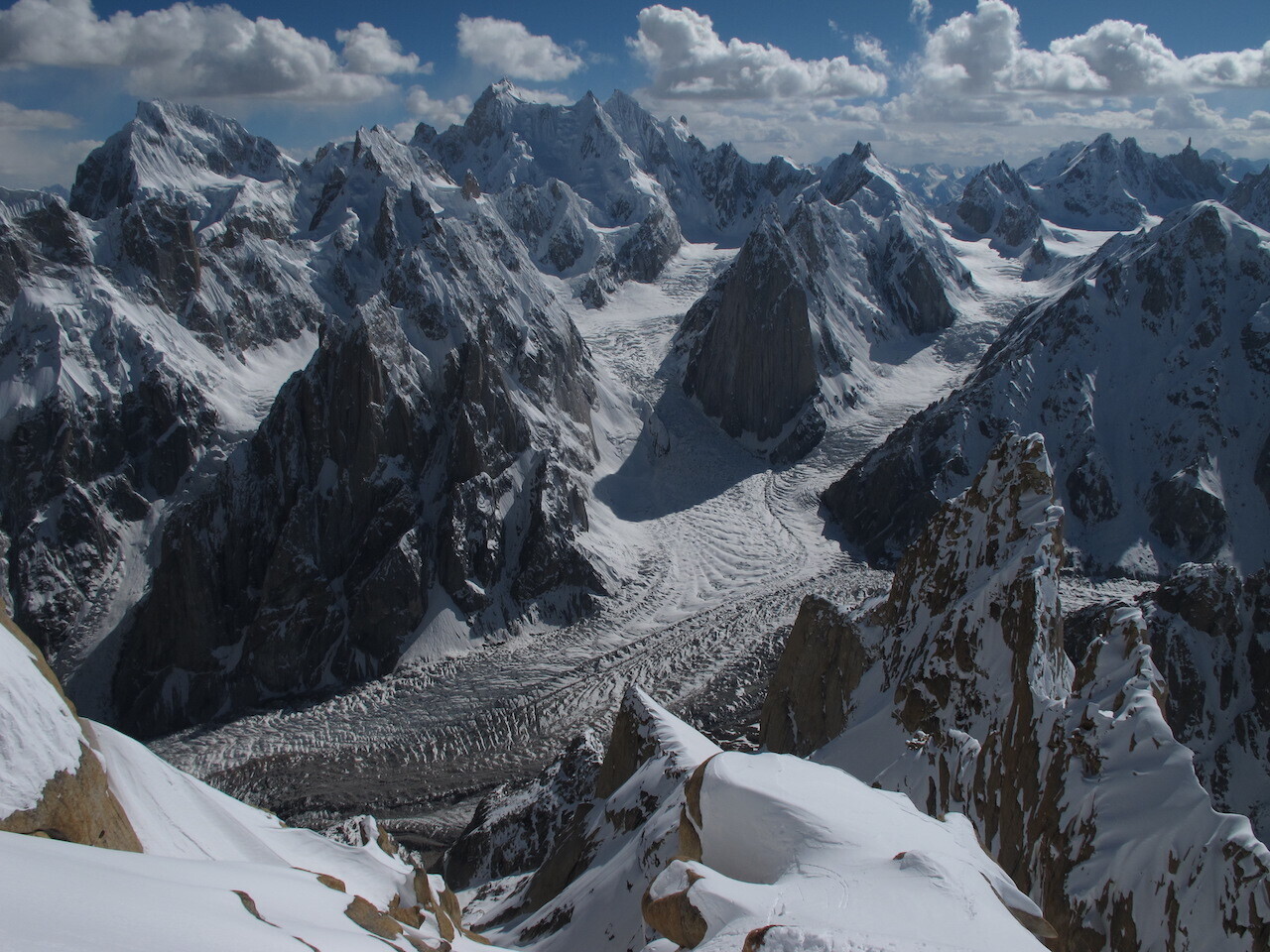
Amendment 42: A.30 Understanding Changes in High Mountain Asia Deferred to ROSES-25
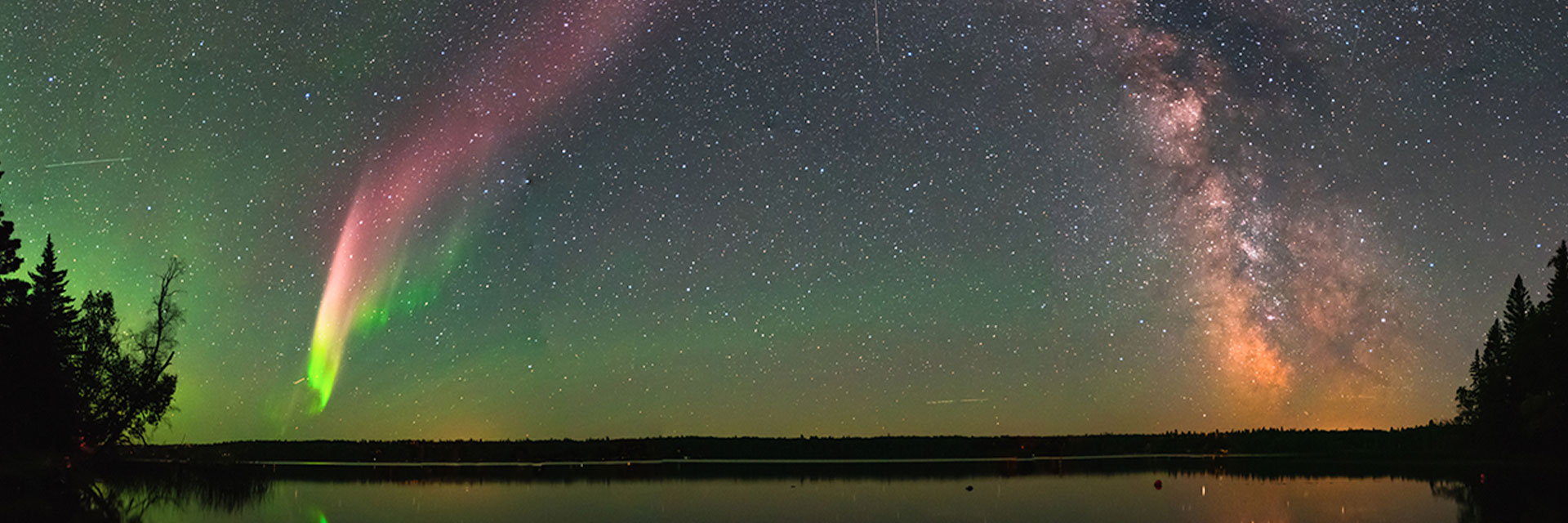
Citizen Science Earth Projects

Solar Eclipse Data Story Helps the Public Visualize the April 2024 Total Eclipse
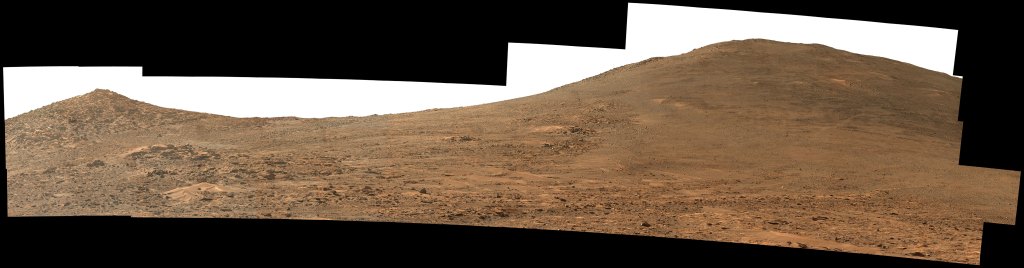
NASA’s Perseverance Rover to Begin Long Climb Up Martian Crater Rim
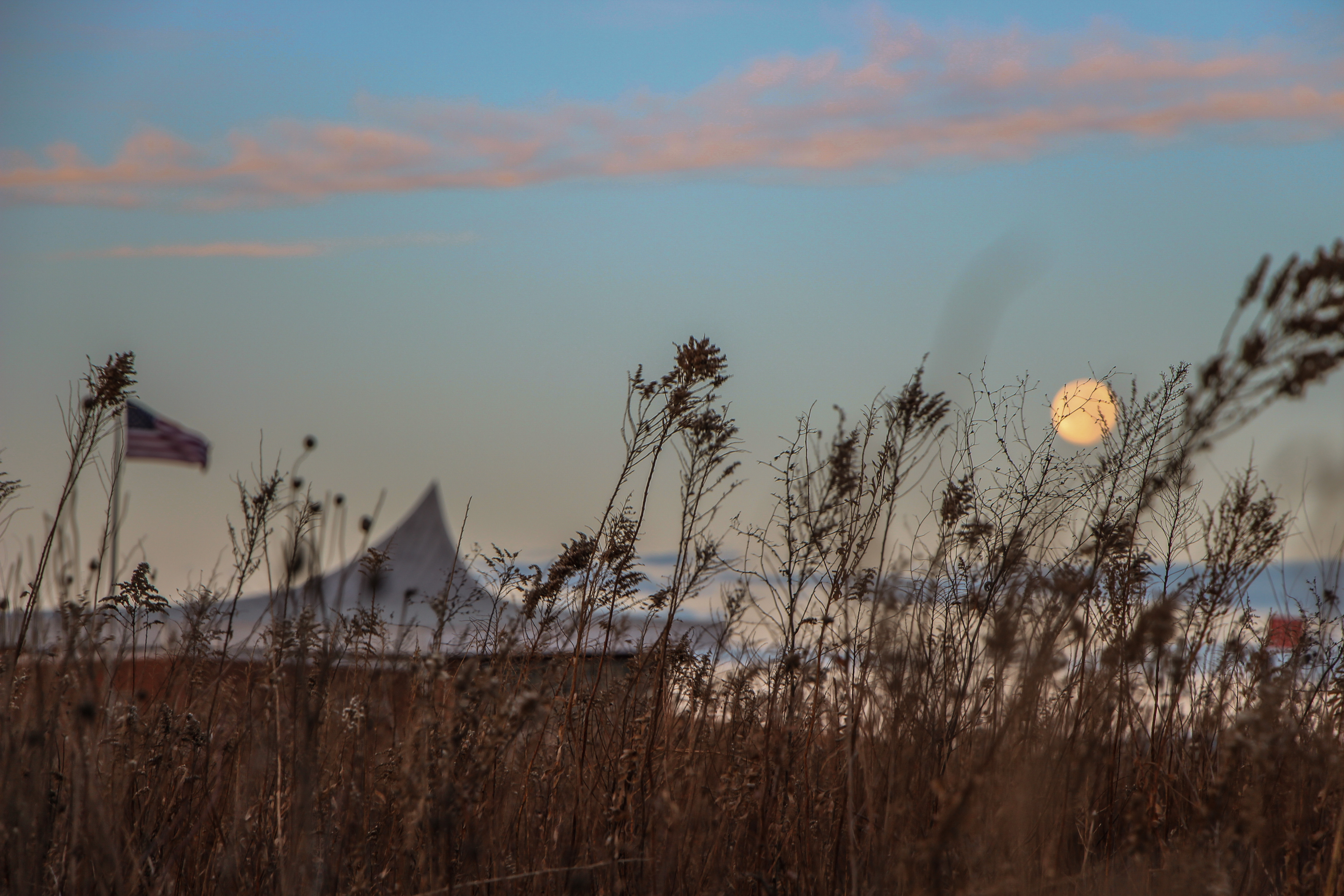
The Next Full Moon is a Supermoon Blue Moon
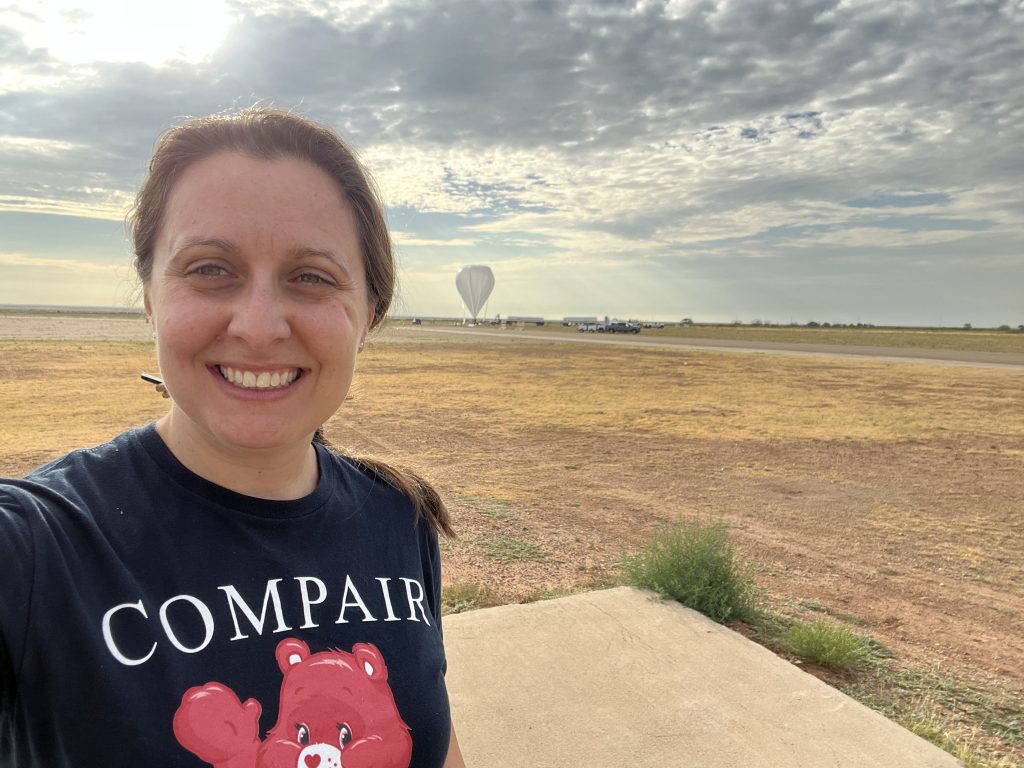
Regina Caputo Charts the Future of High-Energy Astrophysics
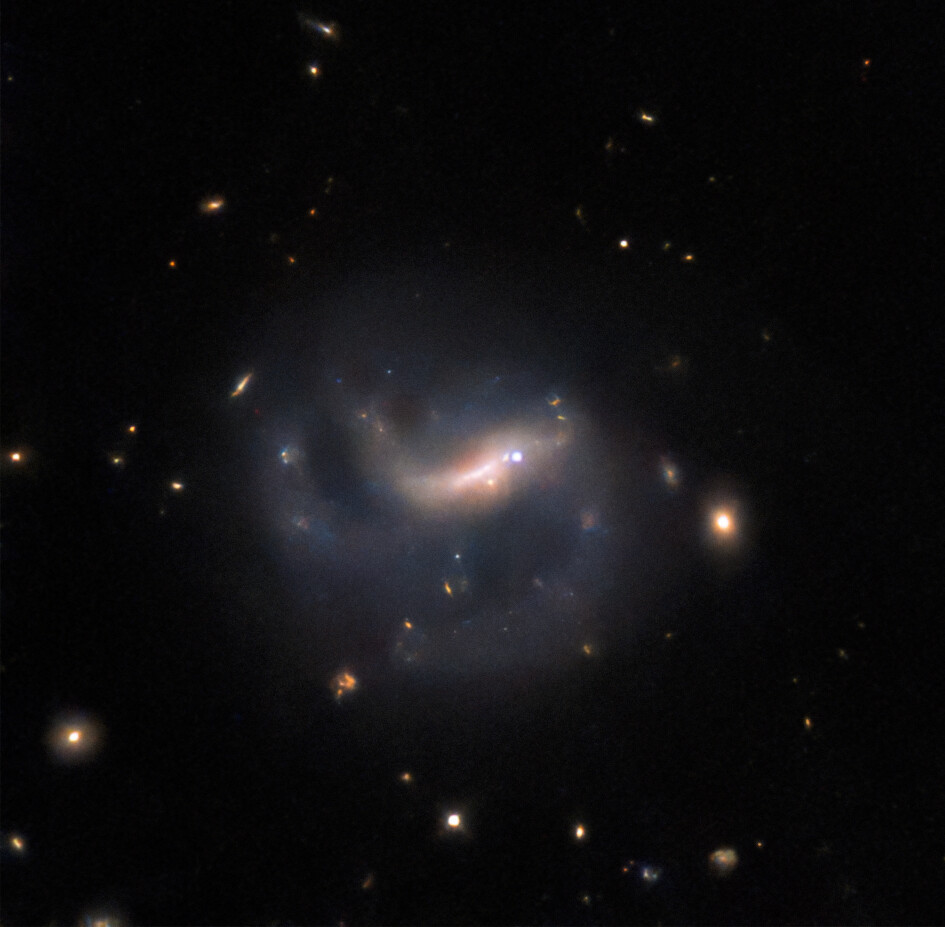
Hubble Spotlights a Supernova
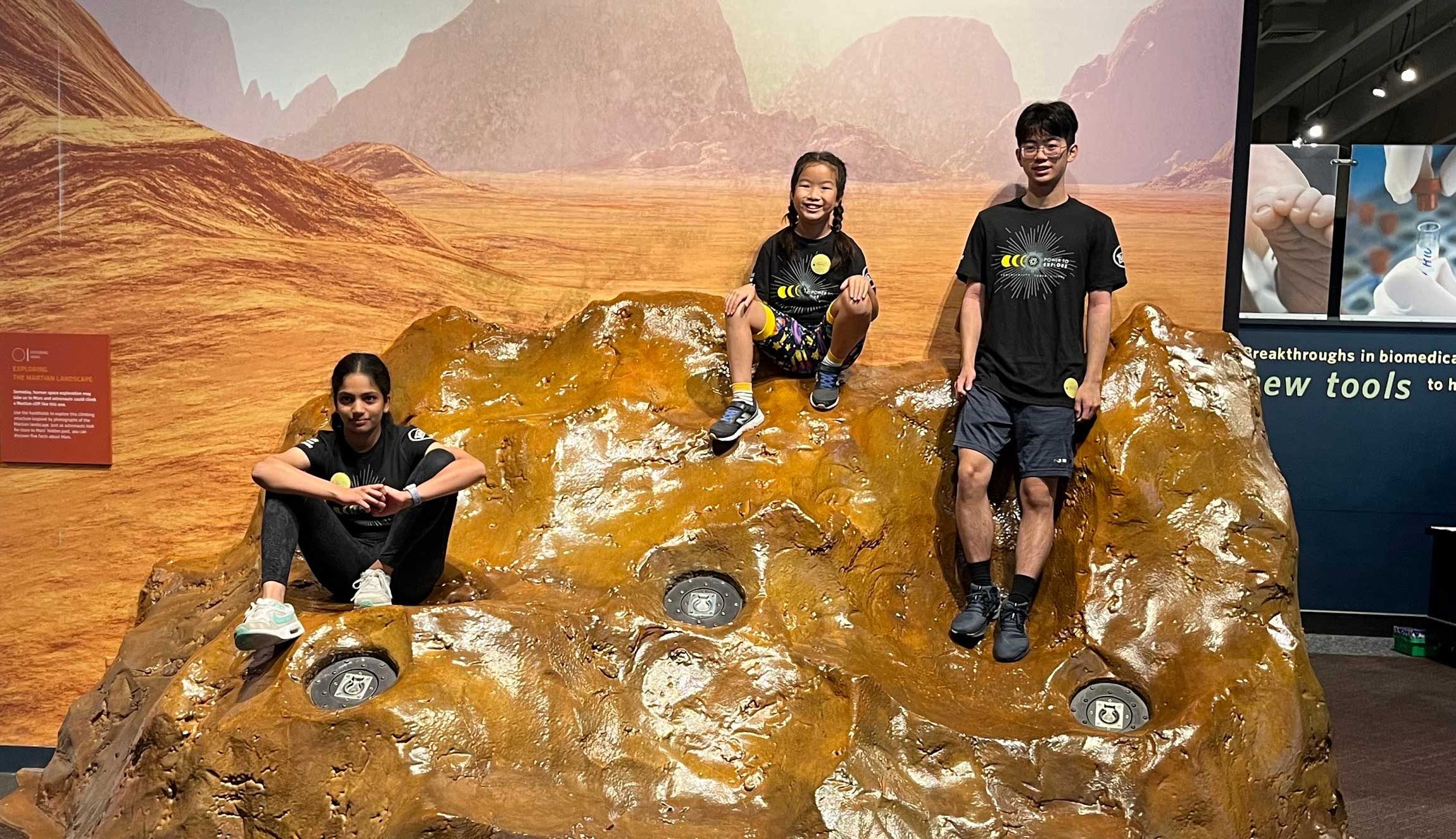
Perseverance Pays Off for Student Challenge Winners
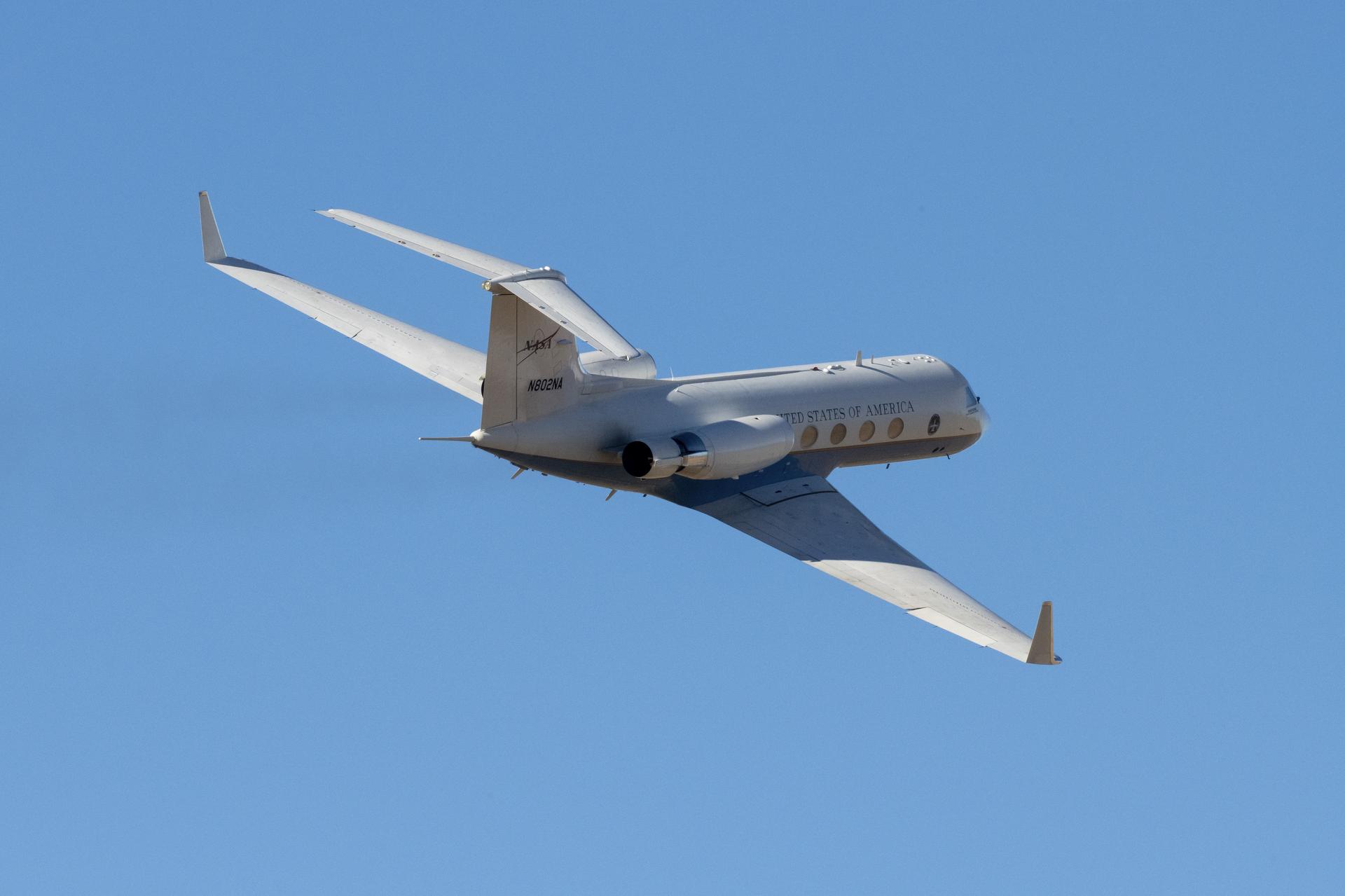
NASA Aircraft Gathers 150 Hours of Data to Better Understand Earth
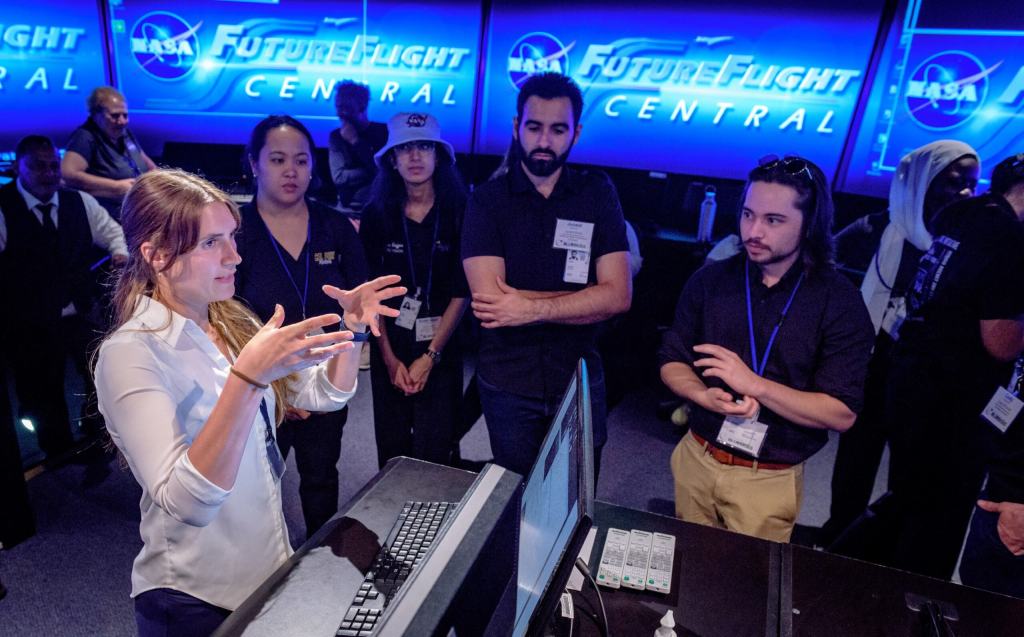
Collegiate Teams to Focus on Aviation Solutions for Agriculture in 2025 Gateways to Blue Skies Competition
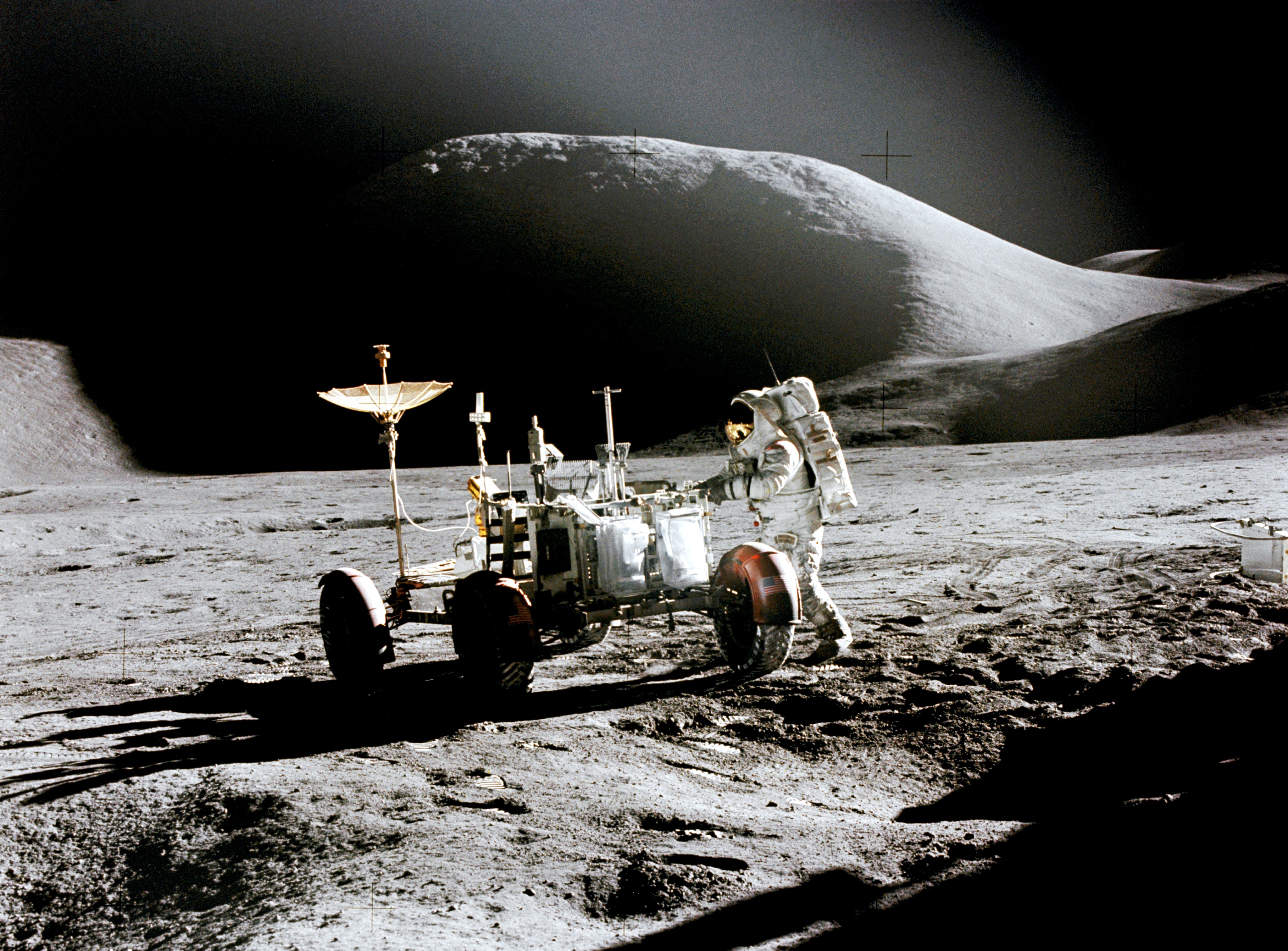
Amendment 41: DRAFT F.13 Lunar Terrain Vehicle Instruments Program Released for Community Comment.
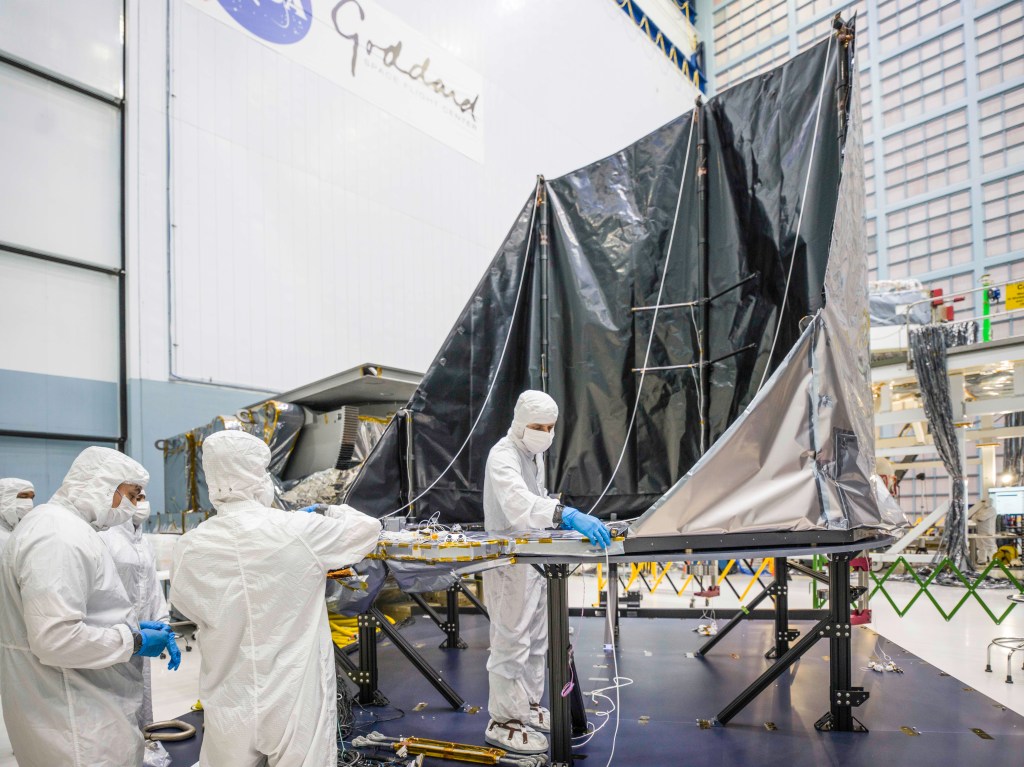
NASA Tests Deployment of Roman Space Telescope’s ‘Visor’

How Do I Navigate NASA Learning Resources and Opportunities?

NASA Challenge Seeks ‘Cooler’ Solutions for Deep Space Exploration
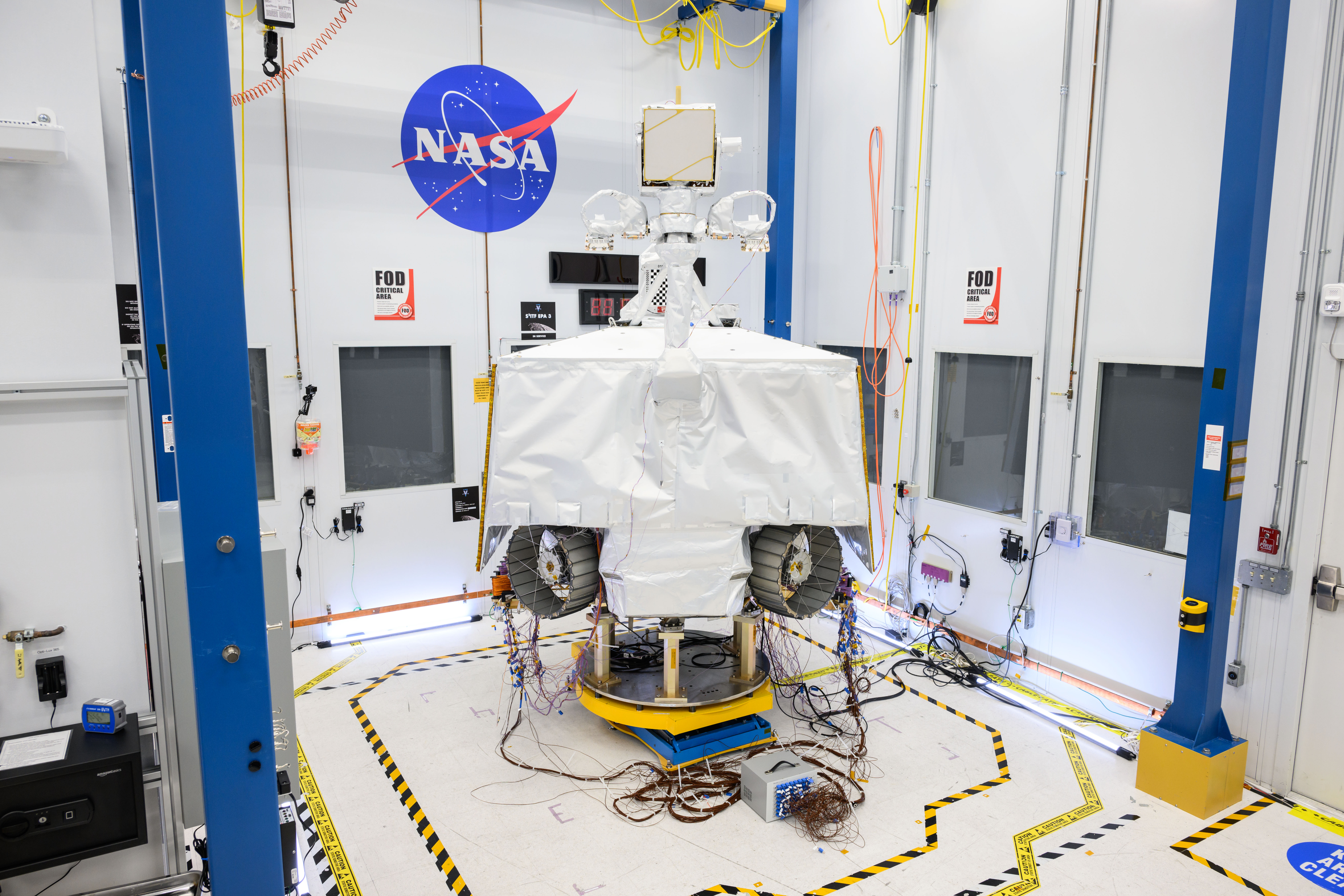
NASA Explores Industry, Partner Interest in Using VIPER Moon Rover
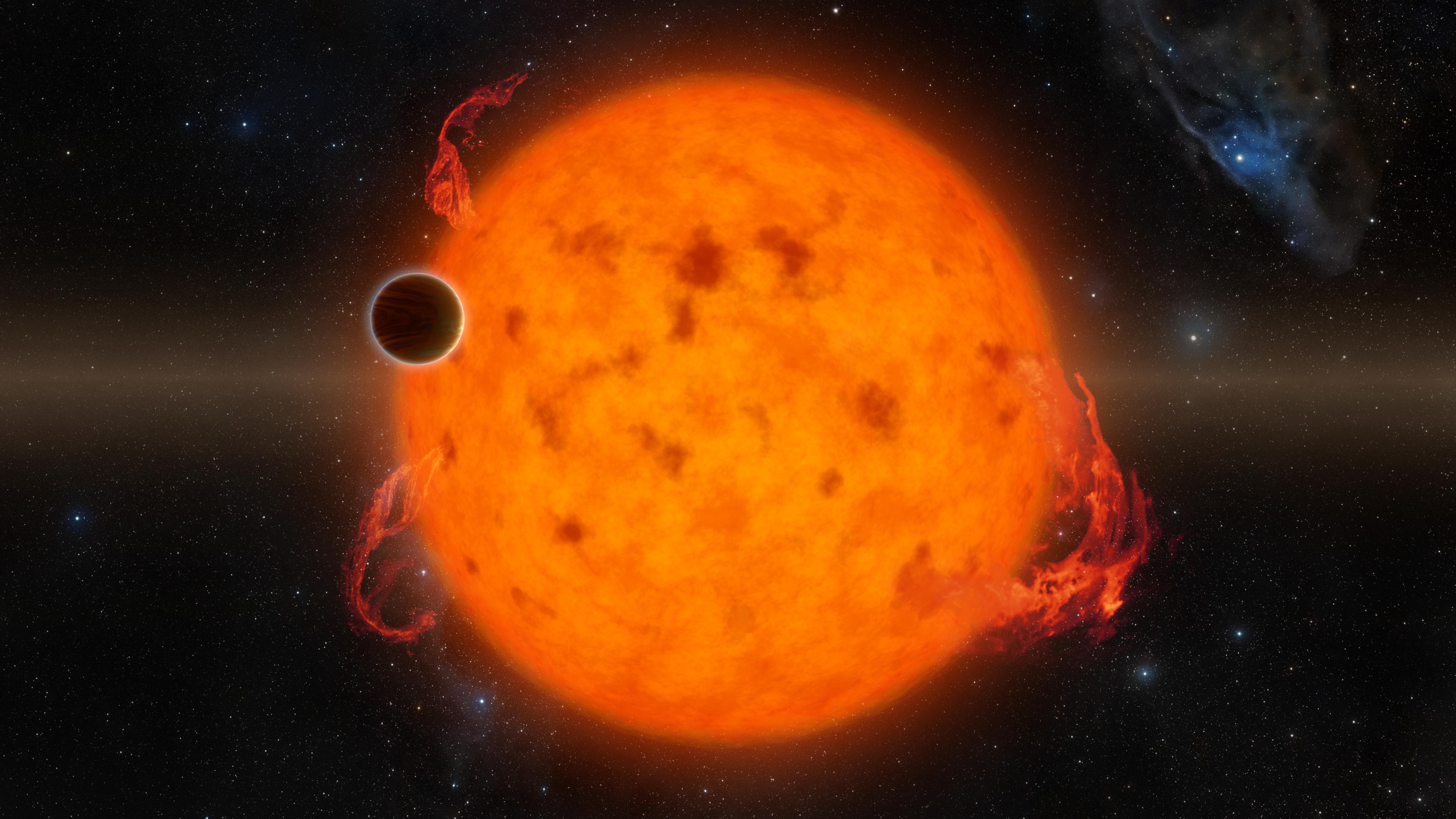
How NASA Citizen Science Fuels Future Exoplanet Research
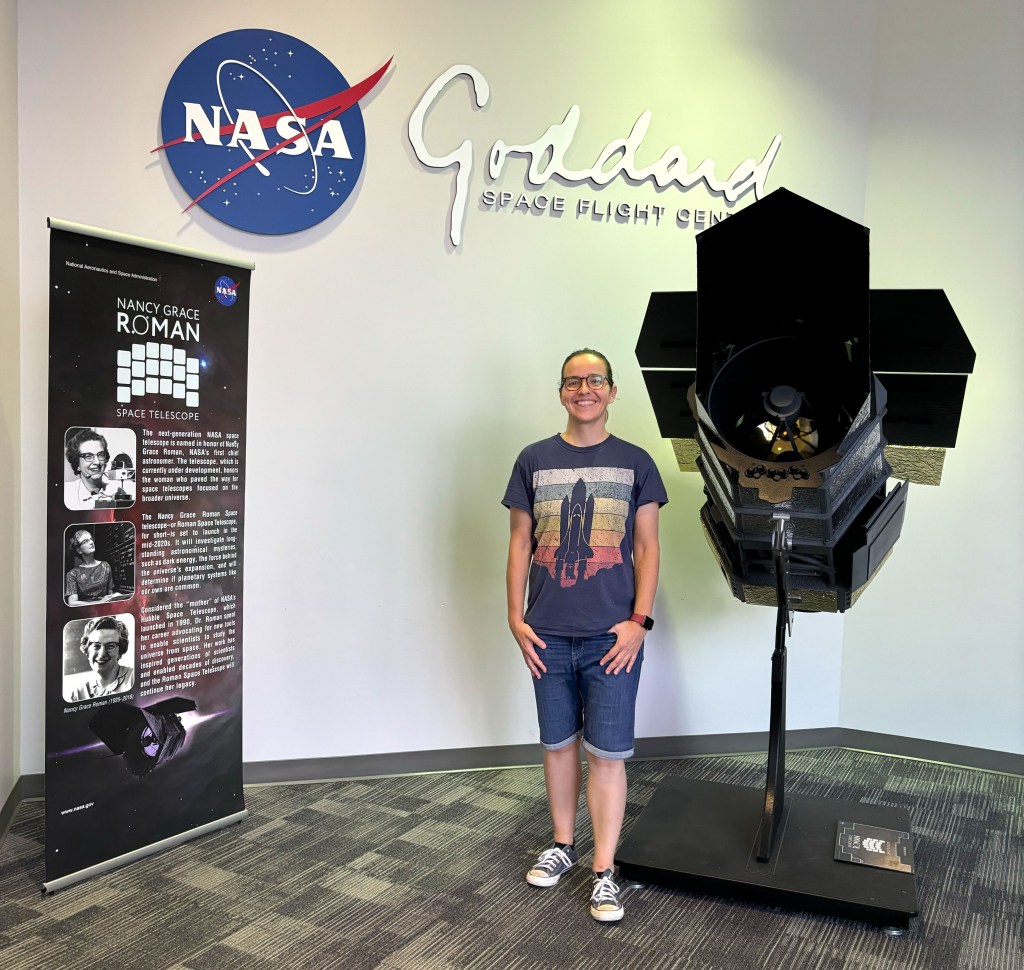
There Are No Imaginary Boundaries for Dr. Ariadna Farrés-Basiana
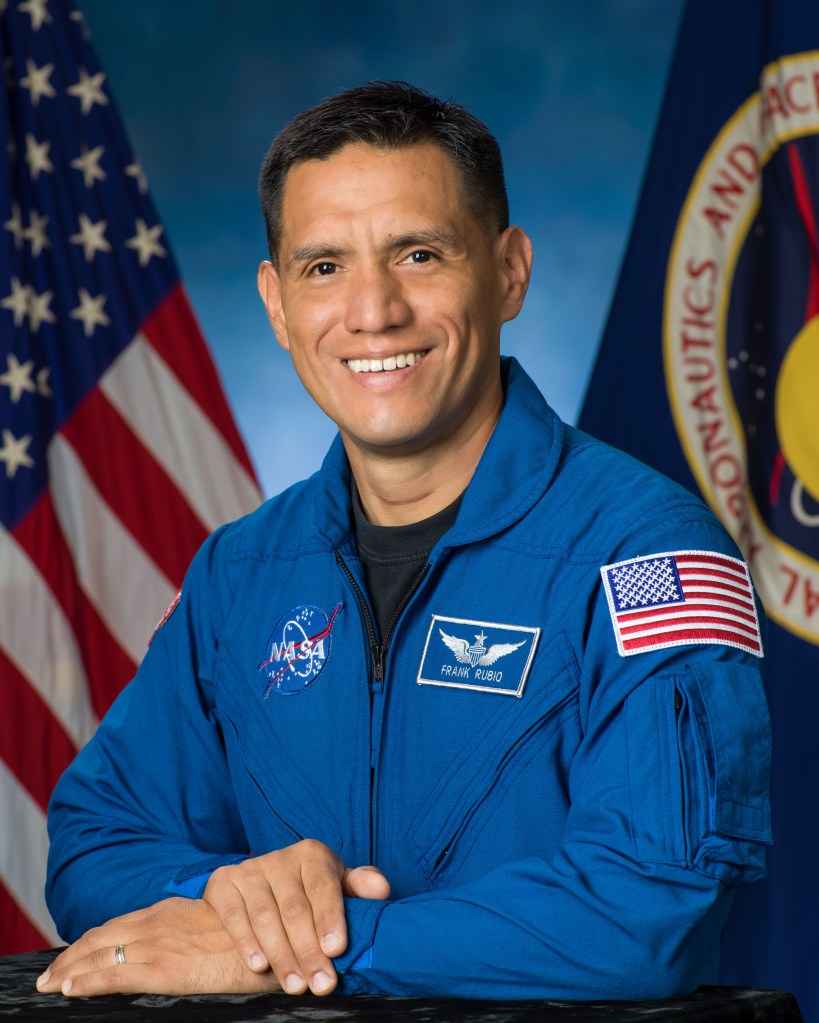
Astronauta de la NASA Frank Rubio
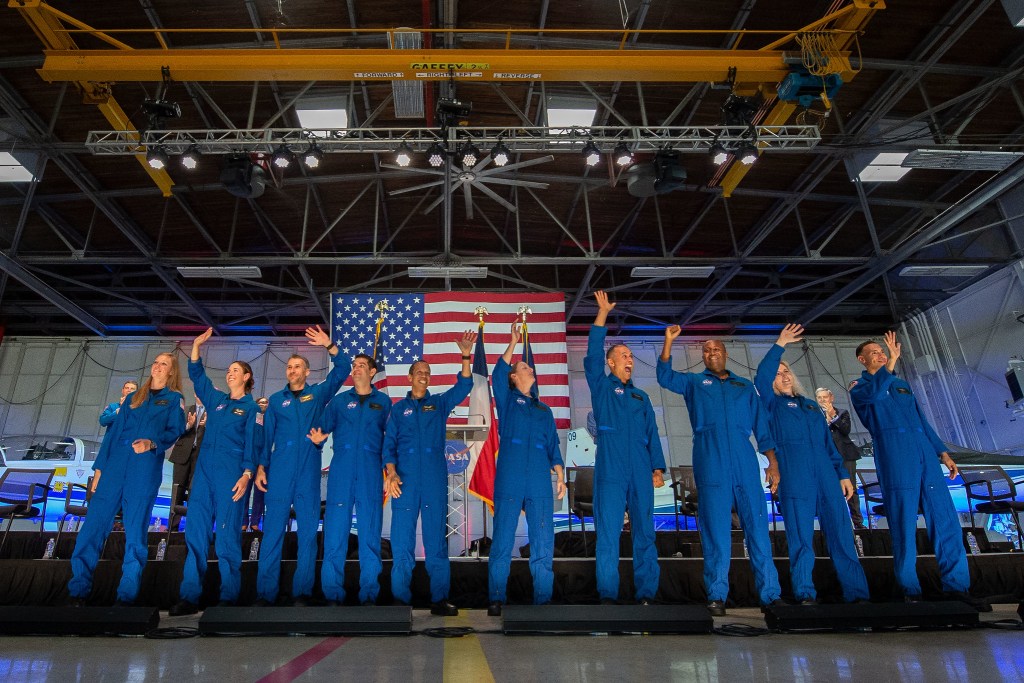
Diez maneras en que los estudiantes pueden prepararse para ser astronautas
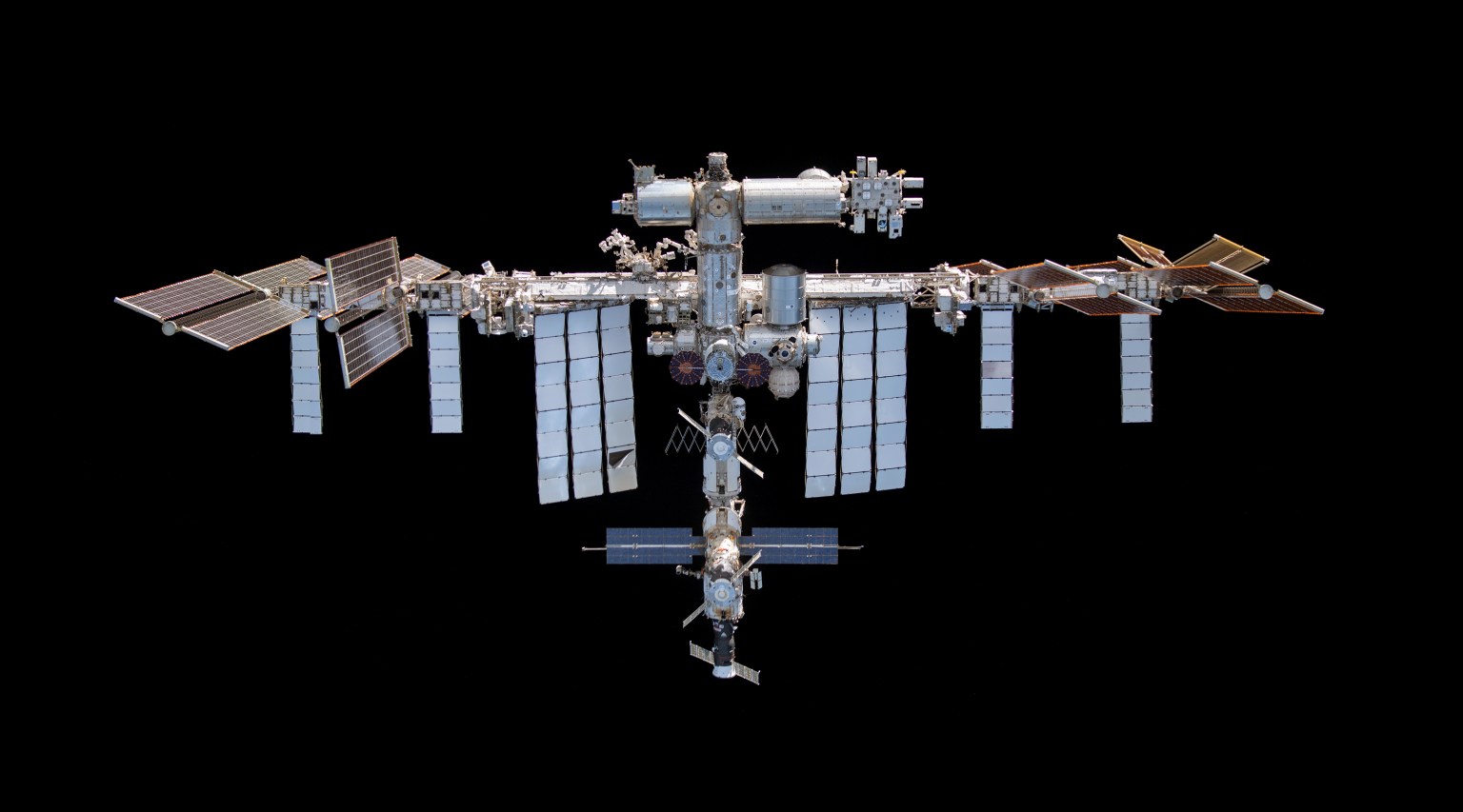
Years in Orbit
Astronauts visited, international space station, about the international space station.
The station was designed between 1984 and 1993. Elements of the station were in construction throughout the US, Canada, Japan, and Europe beginning in the late 1980s.
The International Space Station Program brings together international flight crews, multiple launch vehicles, globally distributed launch and flight operations, training, engineering, and development facilities, communications networks, and the international scientific research community.
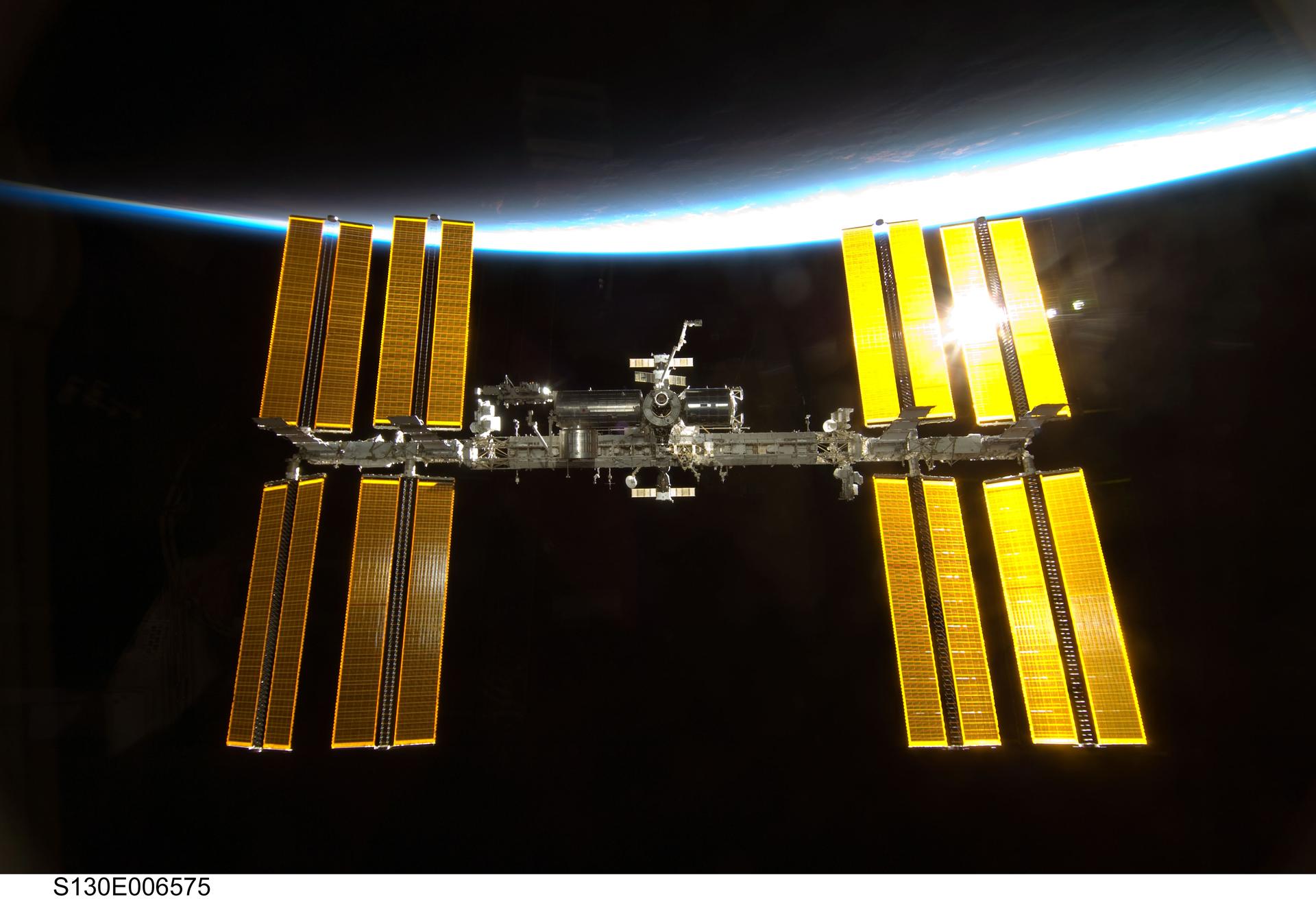
Expedition 71
Expedition 71 began on April 5, 2024 and ends in September 2024. This crew will explore neuro-degenerative diseases and therapies, space botany, space-caused fluid shifts, and algae-based life support systems.
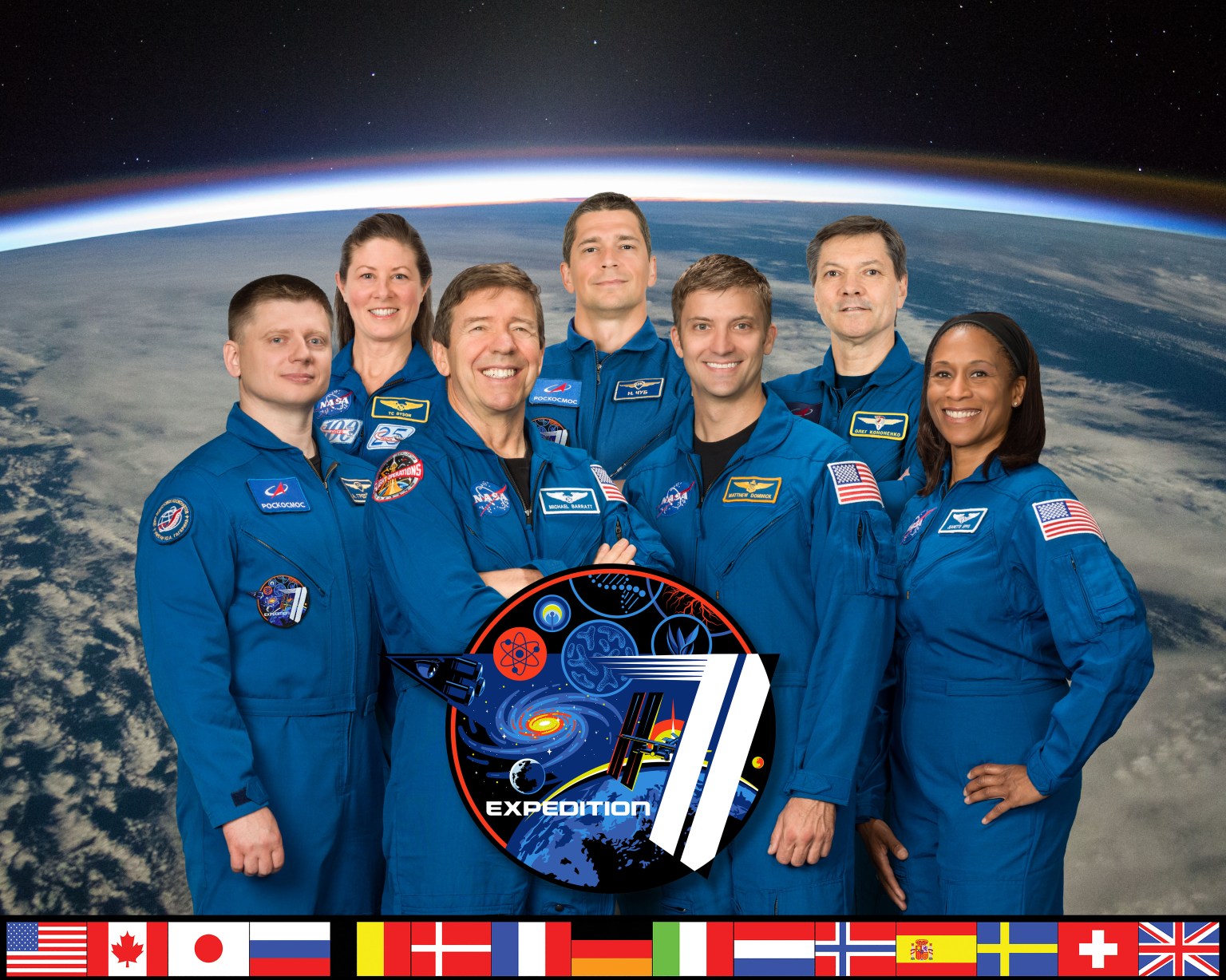
Space Station Daily Blog Update
Daily updates from the orbiting laboratory
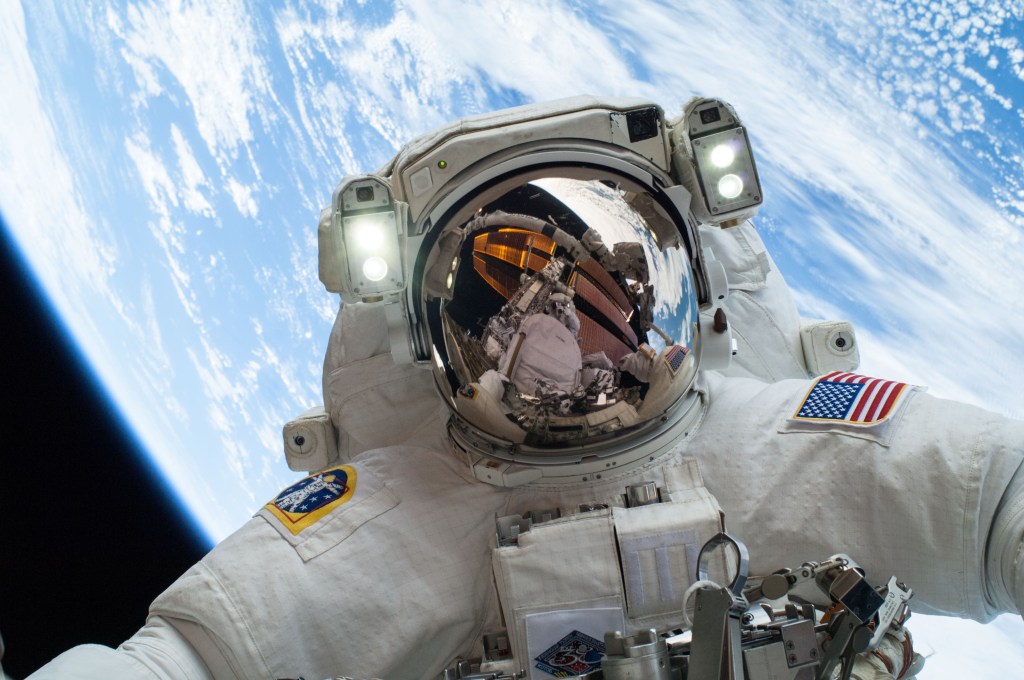
- Assembly Elements
International Cooperation
Facts and figures, station visitors, station record holders, station assembly elements.
Explore the International Space Station.
We are driven to explore the unknown, discover new worlds, push the boundaries of our scientific and technical limits, and then push further.
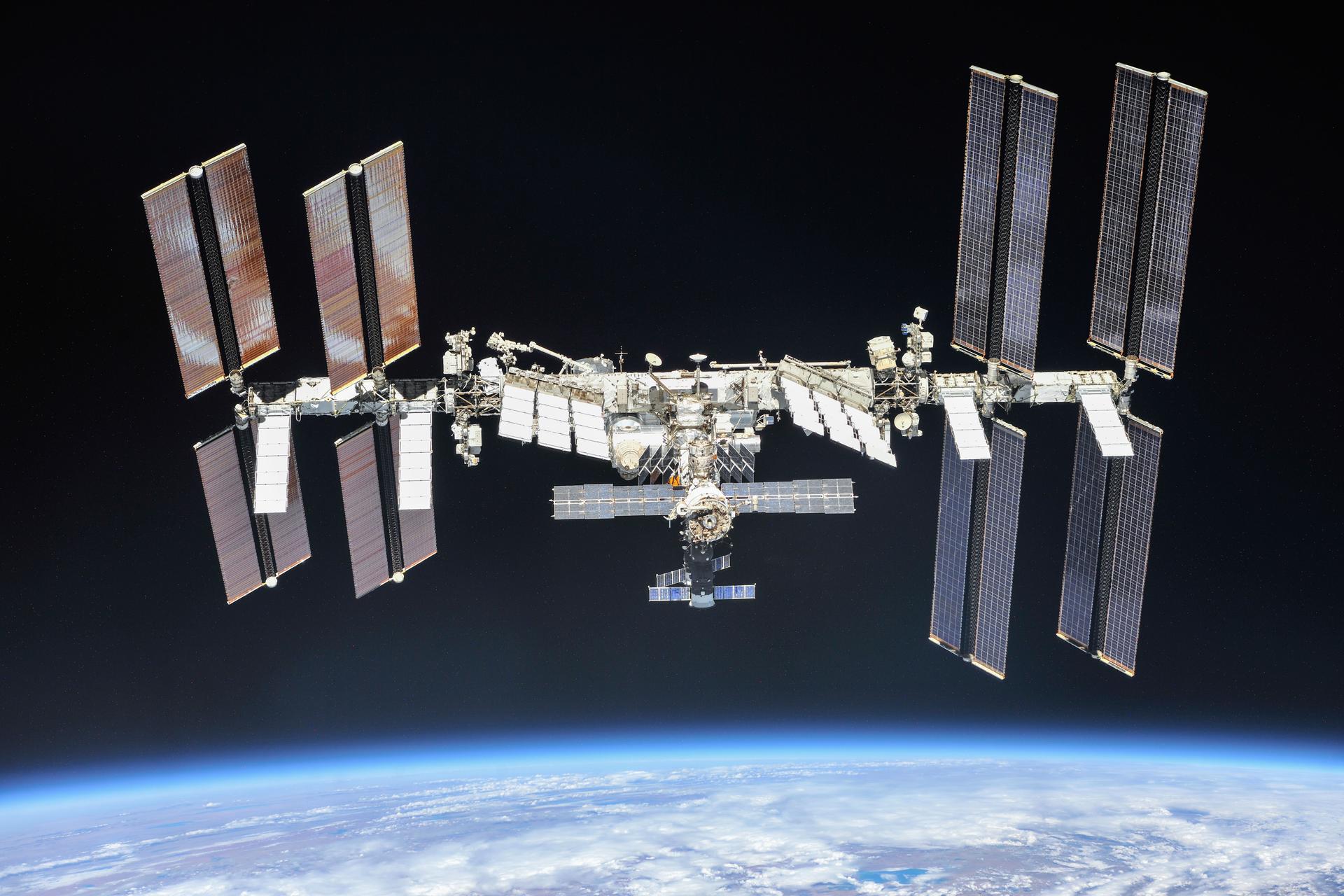
The International Space Station Program’s greatest accomplishment is as much a human achievement as it is a technological one—how best to plan, coordinate, and monitor the varied activities of many organizations and operations.
An international partnership of space agencies provides and operates the elements of the space station. The principals are the space agencies of the United States, Russia, Europe, Japan, and Canada.

Explore quick facts about the International Space Station.
Learn more about the international collaboration, missions, research, and technology that make the space station a unique place.
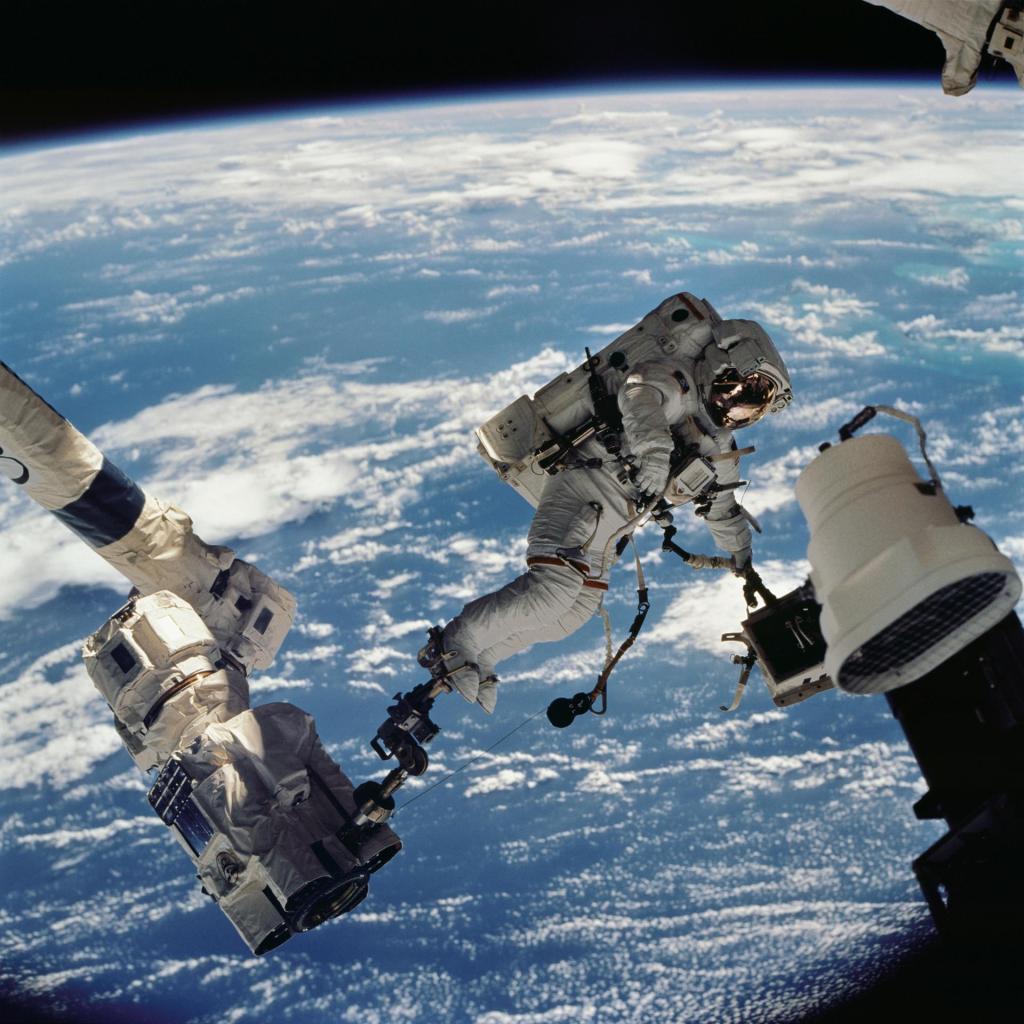
Over 260 individuals representing 20 countries and five International Partners have visited the International Space Station.
Learn more about the people from around the world who have visited the station.

NASA astronauts often spend extended periods of time aboard the International Space Station.
NASA tracks and publishes the single spaceflight record holders (the most days spent in space on a single mission) and the cumulative days in space record holders (combined time in space over an astronaut’s career).

Frequently Asked Questions
Learn more about the orbital complex.
Launched in 1998 and involving the U.S., Russia, Canada, Japan, and the participating countries of the European Space Agency — the International Space Station is one of the most complex international collaborations ever attempted.
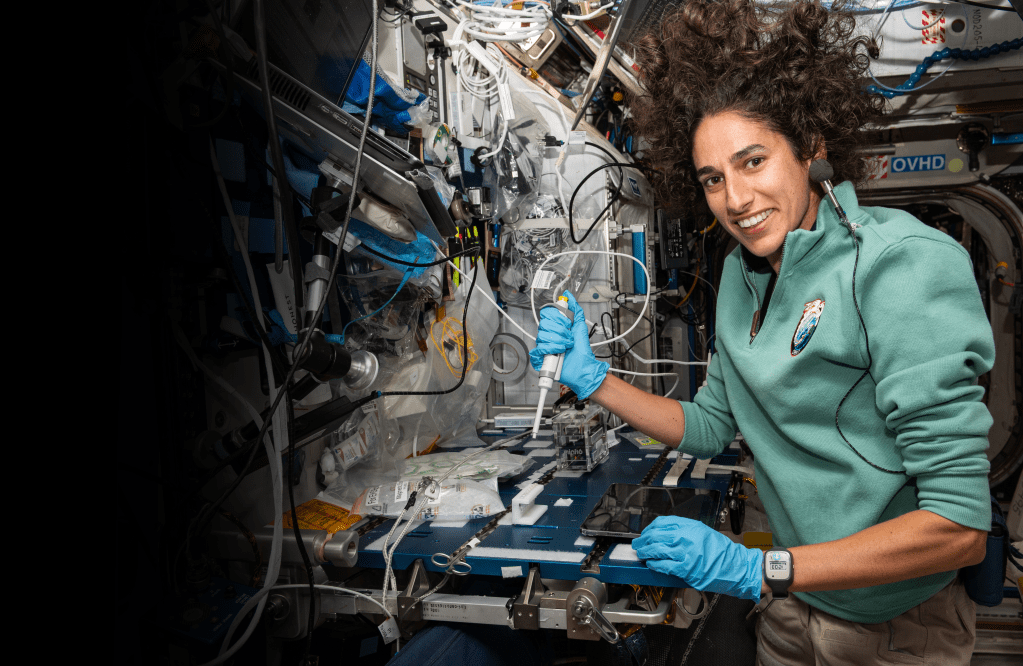
Latest News
NASA to Provide Crew Flight Test Status Update

NASA to Provide Coverage of Progress 89 Launch, Space Station Docking
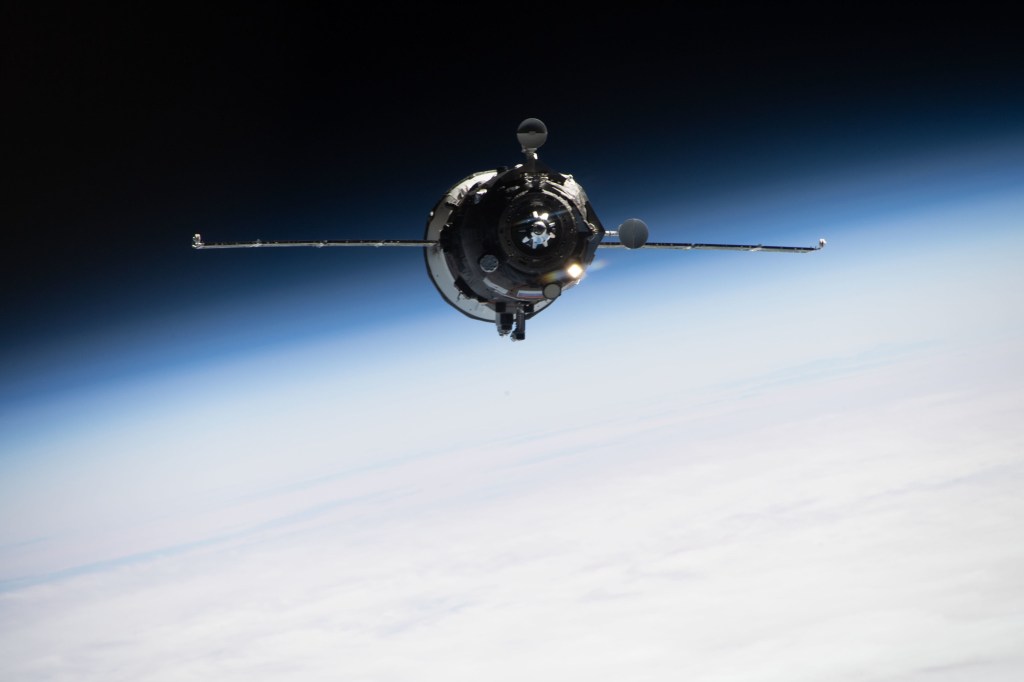
NASA Hosts Astronaut Don Pettit Prelaunch Interviews

NASA to Provide Crew Flight Test, Space Station Missions Update

NASA Science, Cargo Launch on 21st Northrop Grumman Mission to Station
Learn More and Get Involved
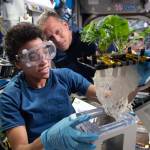
Research and Technology
Since the first crew’s arrival aboard over twenty years ago, the International Space Station has evolved into a state-of-the-art scientific lab.
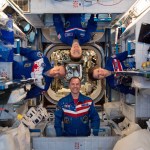
Station Expeditions
Stay up-to-date with the latest content from NASA as we explore the universe and discover more about our home planet.

Visiting Vehicles
ESA, JAXA, Roscosmos, Northrop Grumman, and SpaceX, each have launched their own space freighters to resupply the International Space Station. NASA, Roscosmos, SpaceX, and Boeing have also launched their own crew ships to the orbital outpost.
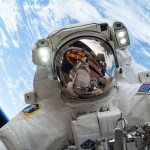
Station Spacewalks
There have been 259 spacewalks at the International Space Station since December 1998.
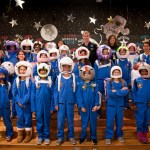
STEM Resources and Opportunities
Explore hands-on activities, interactive, lesson plans, educator guides, and other downloadable content about the International Space Station.
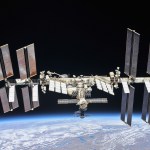
ISS National Laboratory
In an effort to expand the research opportunities of this unparalleled platform, the space station was designated as a U.S. National Laboratory in 2005 by Congress, enabling space research and development access to a broad range of commercial, academic, and government users.
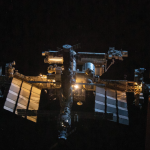
ISS: 2024 Calendar
Explore the new International Space Station 2024 calendar and learn more about the one-of-a-kind orbiting laboratory.
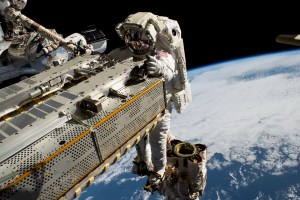
To view more images, visit the Space Station Gallery .
Space station videos.
A YouTube playlist containing a variety of videos about the International Space Station.
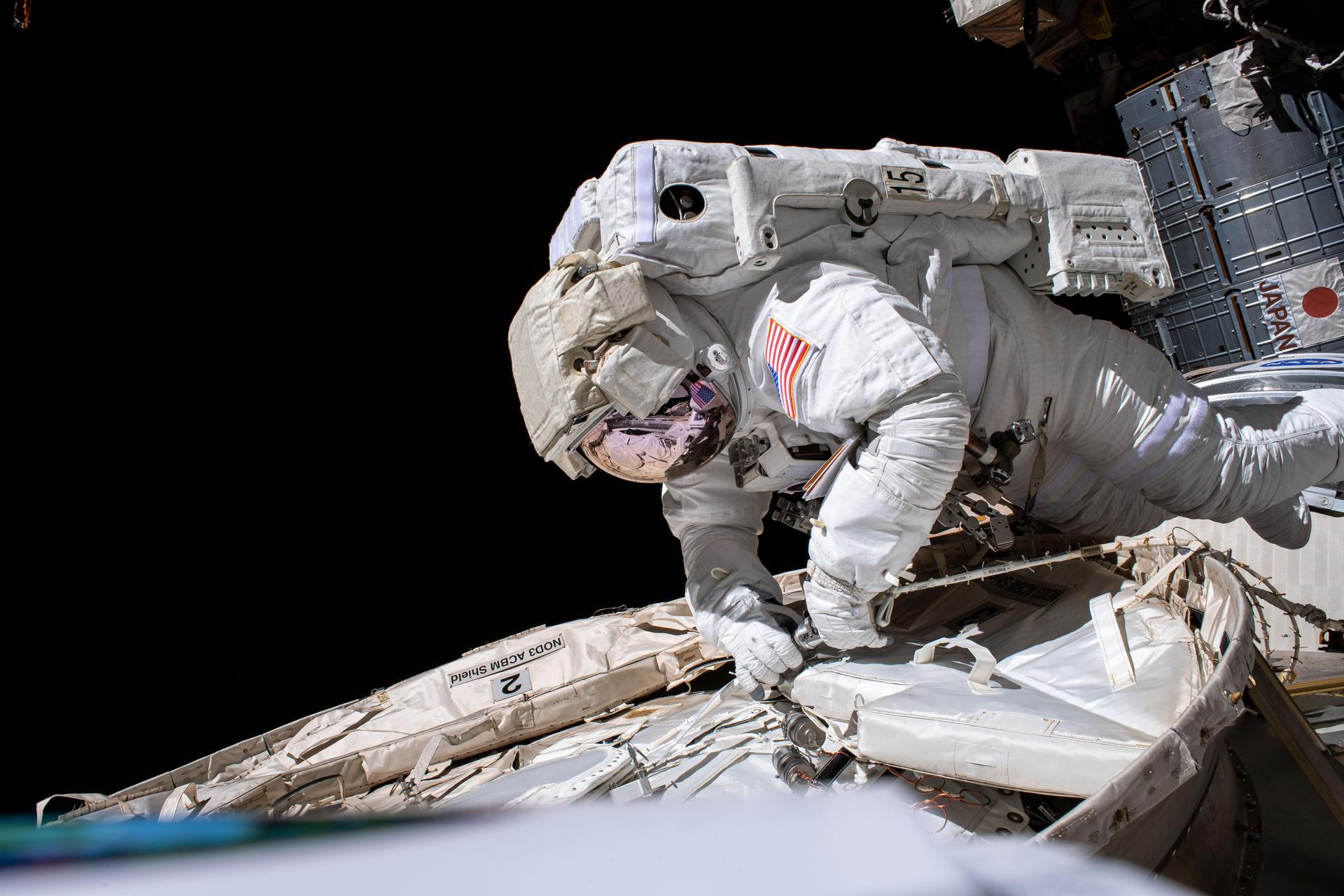
Discover More Topics From NASA
Humans In Space
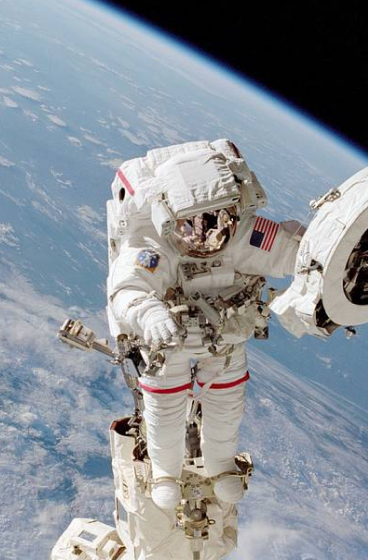
Space Station Research and Technology
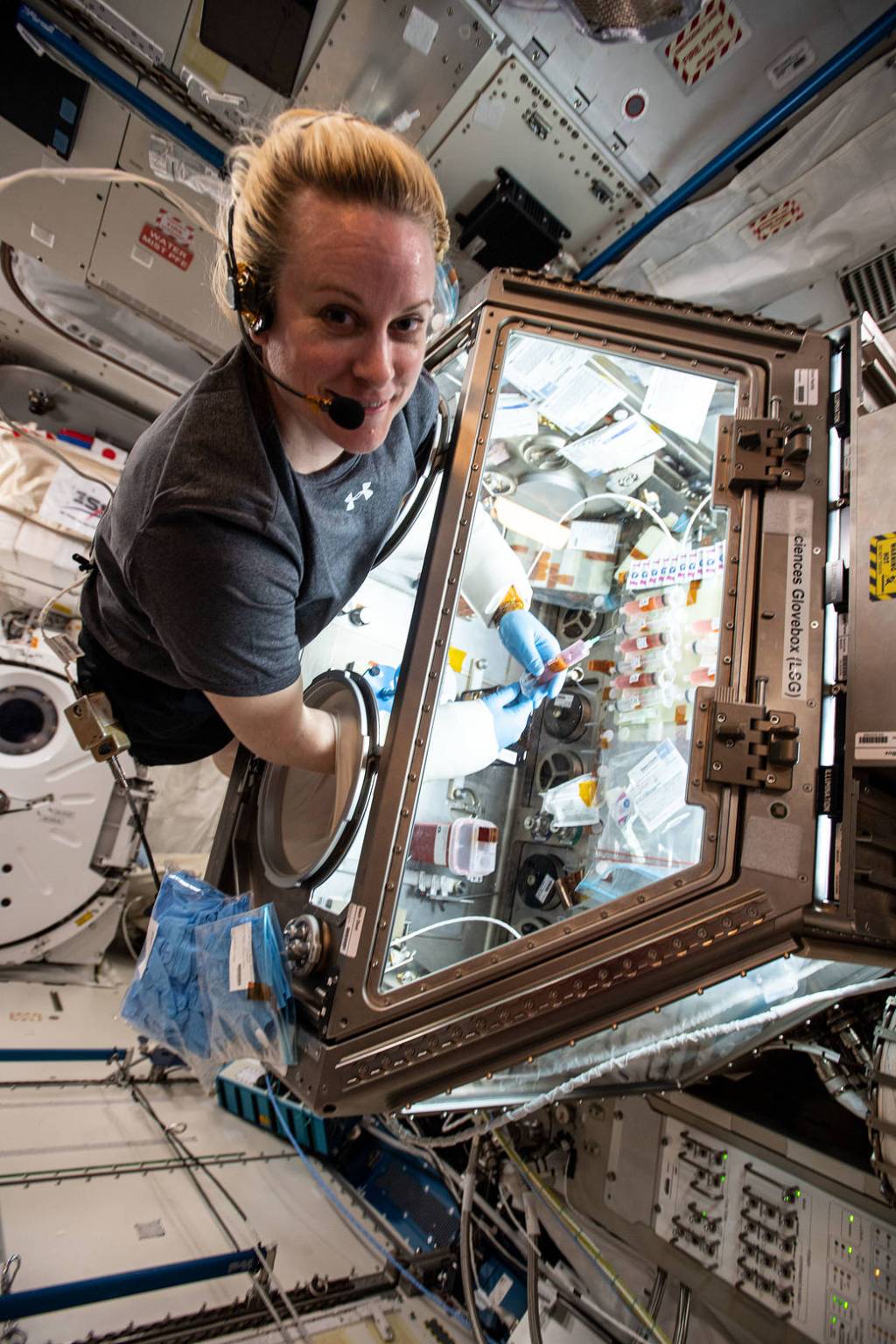
Station Benefits for Humanity
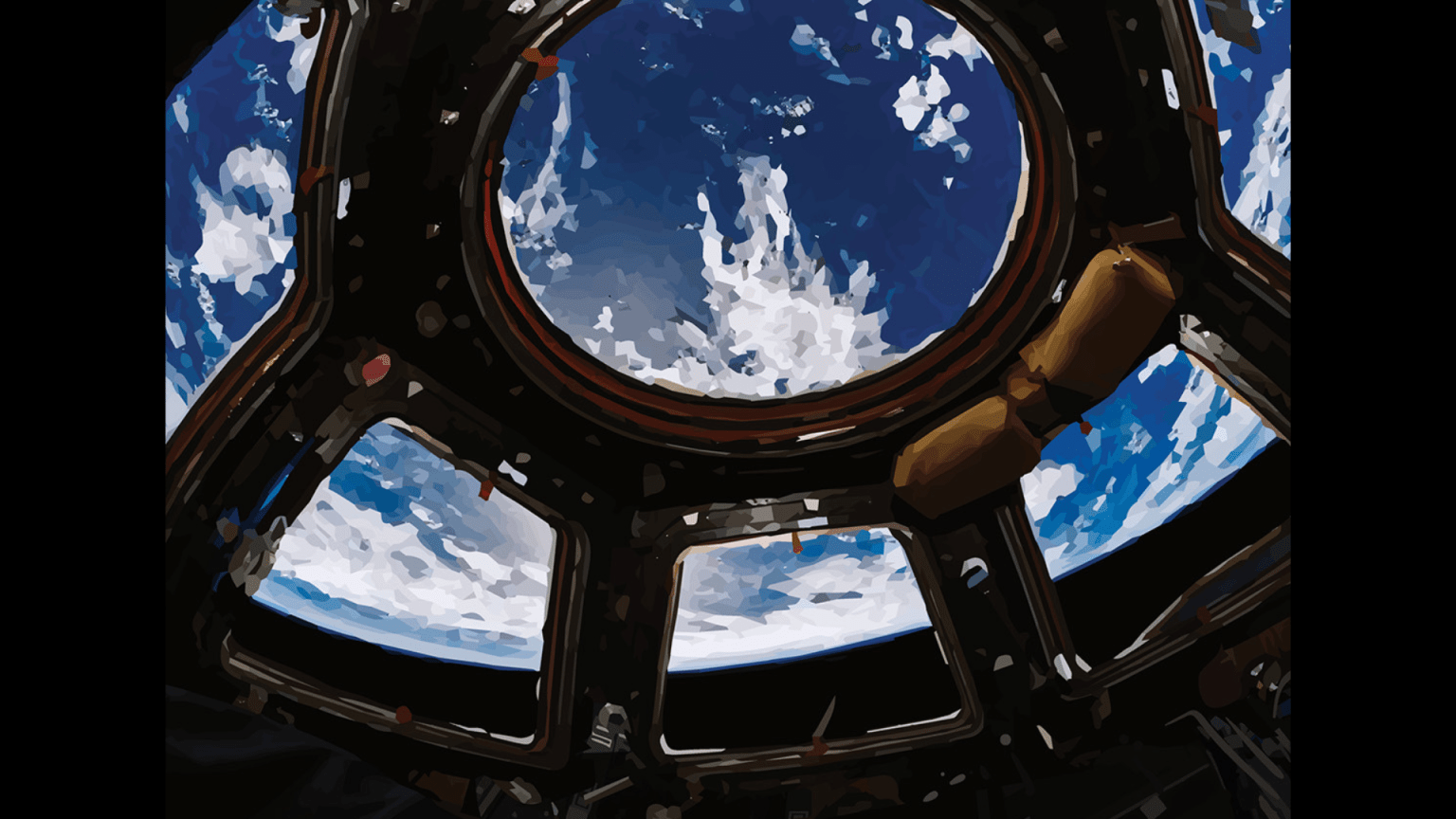
- canva icon Canvas
- Panther Mail (students)
- Arts & Film
- Business & Economy
- Campus & Students
- College Knowledge
- Health & Behavior
- Humanities & Society
- Science & Engineering
- University News
- Chapman Magazine
- Chapman Forward
- Maps & Directions
- Visit Chapman
- Discover Chapman
- Facts & Rankings
- Campus Services
- Degrees & Programs
- Schools & Colleges
- Academic Calendar
- Faculty Directory
- Course Catalogs
- International Study
- Undergraduate Admission
- Undergraduate Application
- Graduate Admission
- Graduate Application
- Affordability
- Financial Aid Calculator
- Campus Tours
- Get Involved
- Career Support
- Diversity & Inclusion
- Fish Interfaith Center
- Health & Safety
- Residence Life
- Student Life
- Pre-Award Administration
- Post-Award Administration
- Research Integrity
- Institutes & Centers
- Center for Undergraduate Excellence
- Graduate Research Support
- Contact Development
- Areas to Support
- Alumni Involvement
- Prospective Students
- Current Students
- Faculty & Staff
- Parents & Families
- All Directories
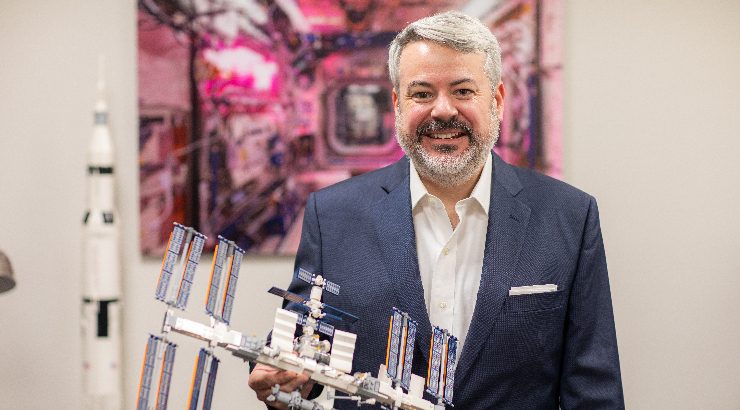
Newly published report outlines findings from first archaeology project in space
The first-ever archeological survey in space has provided new insights into how astronauts use and adapt their living space on the International Space Station, which could influence the design of new space stations after the ISS is decommissioned.
Findings from the research team behind the International Space Station Archaeological Project (ISSAP) were published today in the journal PLOS ONE . Archaeologist Justin Walsh of Chapman University is available to discuss the discoveries of the team’s first on-orbit project, the Sampling Quadrangle Assemblages Research Experiment (SQuARE).
While Earth-bound archaeologists dig one-meter squares to understand a site and strategize further study, the ISSAP team had the astronauts use adhesive tape to define one-meter areas of the International Space Station and document them with daily photographs to study how the spaces were used over 60 days in 2022. The squares were placed in a handful of work and leisure locations on the space station, including the U.S. galley table, workstations, experimental EXPRESS racks and on the wall across from the latrine where astronauts kept their toiletries.
The team’s findings provide the first glimpse into how astronauts adapt to life and conduct research without gravity, how international cooperation plays out in the tight quarters, how they use their space for work and leisure while in orbit, and more. By cross-referencing the photos with astronaut activity reports, the researchers found that the area near the exercise equipment and latrine, while not designated for any particular purpose, had been used as storage for toiletries, resealable bags, and a rarely used computer. The equipment maintenance area was actually used for storage, with little maintenance carried out there.
Beyond informing the future of space habitats, these findings demonstrate how traditional archaeological techniques can be adapted to study extreme and remote habitats, such as Antarctic research stations or the peak of Mt. Everest. ISSAP’s innovative work on SQuARE won awards from the Archaeological Institute of America and the American Anthropological Association in 2023, and the team’s two co-PIs were both named to the Explorers Club 50 Class of 2024 .
“Archaeology is not just about the very distant past,” said Walsh, who is also a co-founder of Brick Moon , a consultancy in space habitat design and use. “It’s about using objects, artifacts, built spaces and architecture as primary evidence for how humans behave, interpret and adapt to the world around them. Archaeology has a place in space.”
About Chapman University Founded in 1861, Chapman University is a nationally ranked private university in Orange, California, about 30 miles south of Los Angeles. Chapman serves nearly 10,000 undergraduate and graduate students, with a 12:1 student-to-faculty ratio. Students can choose from 123 areas of study within 11 colleges for a personalized education. Chapman is categorized by the Carnegie Classification as an R2 “high research activity” institution. Students at Chapman learn directly from distinguished world-class faculty including Nobel Prize winners, MacArthur fellows, published authors and Academy Award winners. The campus has produced a Rhodes Scholar, been named a top producer of Fulbright Scholars and hosts a chapter of Phi Beta Kappa, the nation’s oldest and most prestigious honor society. Chapman also includes the Harry and Diane Rinker Health Science Campus in Irvine. The university features the No. 4 film school and No. 66 business school in the U.S. Learn more about Chapman University: www.chapman.edu .
Media Contact: Molly Thrasher. Director of Public Relations | [email protected] | Mobile: 657-561-4359 Carly Murphy, Public Relations Coordinator | [email protected] | Desk: 714-289-3196 | Mobile: 714-497-9683
Carly Murphy
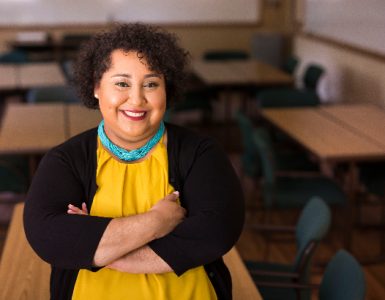
Media Contacts
Newsroom site, chapman site, services & policies.

© 2023 Chapman University
- Arts & Film
- Business & Economy
- Campus & Students
- Health & Behavior
- Humanities & Society
- Science & Engineering
Your Header Sidebar area is currently empty. Hurry up and add some widgets .
- Communication Systems
- Engineering
- Communication Engineering
- Optical Engineering
- Satellite Communication
Satellite Communications in the New Space Era: A Survey and Future Challenges
- February 2020

- University of Luxembourg

- This person is not on ResearchGate, or hasn't claimed this research yet.

Abstract and Figures

Discover the world's research
- 25+ million members
- 160+ million publication pages
- 2.3+ billion citations

- Yannick Poirier

- Frederic Roelens

- Recruit researchers
- Join for free
- Login Email Tip: Most researchers use their institutional email address as their ResearchGate login Password Forgot password? Keep me logged in Log in or Continue with Google Welcome back! Please log in. Email · Hint Tip: Most researchers use their institutional email address as their ResearchGate login Password Forgot password? Keep me logged in Log in or Continue with Google No account? Sign up
NTRS - NASA Technical Reports Server
Available downloads, related records.

COMMENTS
Between Oct. 1, 2021 and Sept. 30, 2022, researchers published more than 400 scientific papers based on studies conducted aboard the space station. Read highlights of some of the groundbreaking space station science published this past year below. For more space station research achievements and additional information about the findings ...
The International Space Station is a microgravity research lab hosting groundbreaking technology demonstrations and scientific investigations. More than 3,700 investigations conducted to date have generated more than 4,000 research articles published in scientific journals. In 2023, the orbiting lab hosted approximately 500 investigations.
The International Space Station is a microgravity research lab hosting groundbreaking technology demonstrations and scientific investigations. More than 3,700 investigations conducted to date have generated more than 4,000 research articles published in scientific journals. In 2023, the orbiting lab hosted approximately 500 investigations.
Over that time, astronauts on the ISS have conducted around 3,000 science experiments. The research spans a range of disciplines, including fundamental physics, Earth observation and biomedical ...
Space is the final frontier for understanding how extreme environments affect human physiology. Following twin astronauts, one of which spent a year-long mission on the International Space Station, Garrett-Bakelman et al. examined molecular and physiological traits that may be affected by time in space (see the Perspective by Löbrich and Jeggo). ). Sequencing the components of whole blood ...
The International Space Station (ISS) is a multi-nation project, the single largest structure ever put in Earth's orbit. It has hosted over 250 people from over 20 countries and over 3000 ...
BPS currently receives $85 million per year, less than 3% of the $3.2 billion allocated to NASA's planetary science division. To support the needed base of scientific researchers, the panel estimates that BPS will need roughly $1 billion a year before the decade's end. The report coincides with a sea change in human spaceflight capabilities.
Space experiments are a technically challenging but a scientifically important part of astrobiology and astrochemistry research. The International Space Station (ISS) is an excellent example of a ...
iting ISS research impact by quantifying ISS research output or its perceived benefits for humanit. .This paper proposes a new assessment of ISS impact from the perspective of the end users' needs. To that end, the authors use visualizations and metrics of scientific publication data to show the ISS research infl.
1. Research on the International Space S tation - An Overview 1. Cynthia A. Evans and Julie A. Robinson. NASA Johnson Space Center, Houston, TX 77058. Judy M. Tate-Brown. Engineering and Science ...
The International Space Station (ISS) is an orbiting laboratory that provides an ideal facility to conduct long-duration experiments in the near absence of gravity and allows continuous and interactive research similar to Earth-based laboratories. This enables scientists to pursue innovations and discoveries not currently achievable by other means.
An archaeologist working on a shovel test pit. Samantha Sanft and Kurt Jordan, CC BY-ND. Most recently, we collected data about how crew used objects inside the space station by adapting one of ...
Mir was the first space station for human's long term presence in space, and it took about ten years to assemble the individual specialized modules after its launch in 1986 [3]. ... There are not many papers on GNC research for space habitats. ISS is a good reference, an overview of ISS GNC systems including state and attitude determination, ...
Between January and March 2022, crew aboard the International Space Station (ISS) performed the first archaeological fieldwork in space, the Sampling Quadrangle Assemblages Research Experiment (SQuARE). The experiment aimed to: (1) develop a new understanding of how humans adapt to life in an environmental context for which we are not evolutionarily adapted, using evidence from the observation ...
The ISS National Lab manages all non-NASA research and investigations to expand research opportunities of this unparalleled platform. Through the ISS National Lab, this unique space-based research platform is available to U.S. researchers from small companies, research institutions, Fortune 500 companies, government agencies, and others, all interested in leveraging the space environment to ...
This paper will summarize science results and accomplishments, and discuss how the early science utilization provides the foundation for continuing research campaigns aboard the ISS that will benefit future exploration programs. This paper is derived from forthcoming NASA/TP-2009-213146-REVISION A4.
Indeed, the ISS "has operated as a bastion of international cooperation and a unique testbed for microgravity research," write MIT engineers in a paper they presented on March 8 at the IEEE Aerospace Conference in Montana. But the ISS will eventually be retired in its current form.
Archaeology in space: The Sampling Quadrangle Assemblages Research Experiment (SQuARE) on the International Space Station. Report 1: Squares 03 and 05 . PLOS ONE , 2024; 19 (8): e0304229 DOI: 10. ...
In response to the US President's Vision for Space Exploration (January 14, 2004), NASA has revised its utilization plans for International Space Station (ISS) to focus on (1) research on ...
REE biomining in space. Data were acquired using the BioRock biomining reactor, designed for these experiments (Fig. 1) which contained basaltic rock with known REE composition (Table 1) and major ...
Abstract. The Bharatiya Antariksha Station, colloquially known as the Indian Space Station (ISS), symbolizes India's ambitious foray into space exploration. Proposed and operated by the Indian ...
In the past, ISS Program Science Forum members' publications on the impact of the space station have covered subjects such as Expanded benefits for humanity from the International Space Station [1], ISS research results and accomplishment output [2], Benefits of International Collaboration of the ISS [3], assessing ISS benefits for humanity ...
A detailed explanation of the requirement, operation, and construction of NASA's "Robonauts" designed for the International Space Station is discussed in the research. The paper also put light on the ATLAS, an asteroid detecting system. The methods for providing medical aid to the crew, debris and its influence, analyzing data and ...
The International Space Station National Laboratory, in partnership with NASA's Biological and Physical Sciences division, jointly announced the selection of five projects through the inaugural Igniting Innovation solicitation for cancer and other disease-related research and technology development on station. The projects, which were ...
The study demonstrated the value of archaeological methods in understanding life in space, potentially opening up a new field of space archaeology. Funding & Disclosures. The study was funded by Chapman University's Office of Research and Sponsored Programs. The International Space Station National Laboratory provided access to the ISS and ...
Since 2013, research has been underway on the International Space Station (ISS) National Lab with astronauts conducting experiments in microgravity to ultimately help improve our lives here on Earth.
About the International Space Station. The station was designed between 1984 and 1993. Elements of the station were in construction throughout the US, Canada, Japan, and Europe beginning in the late 1980s. The International Space Station Program brings together international flight crews, multiple launch vehicles, globally distributed launch ...
Findings from the research team behind the International Space Station Archaeological Project (ISSAP) were published today in the journal PLOS ONE. Archaeologist Justin Walsh of Chapman University is available to discuss the discoveries of the team's first on-orbit project, the Sampling Quadrangle Assemblages Research Experiment (SQuARE).
Steven Kisseleff, Jor ge Querol, Lei Lei, Thang X. V u, George Goussetis. Abstract —Satellite communications (SatComs) have recently. entered a period of renewed interest motivated by ...
The Heliophysics Flight Opportunities in Research and Technology (H-FORT) program performs space and science and science-enabling investigations that use platforms including Small Satellites, CubeSats, Hosted Rideshare Payloads, and International Space Station (ISS) attached payloads. The H-FORT program encourages the development of technologies that will enable investigation of heliophysics ...