- Corpus ID: 186202081

KITCHEN WASTE COMPOSTING: A SUSTAINABLE WASTE MANAGEMENT TECHNIQUE
- N. Shukla , S. K. Juneja
- Published 2016
- Environmental Science
Tables from this paper
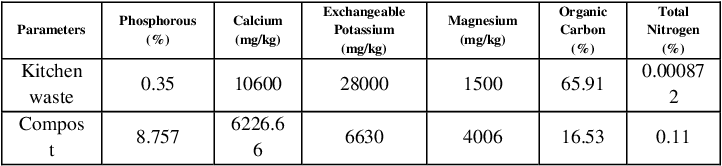
2 Citations
Biogas production from kitchen waste, illustrating china's journey to balance, circular, and secure potassium cycles in the last three decades, 9 references, agricultural and ecological aspects of a sandy soil as affected by the application of municipal solid waste composts, impact of composting strategies on the treatment of soils contaminated with organic pollutants., long-term effects of municipal solid waste compost application on soil enzyme activities and microbial biomass, compost and vermicompost as nursery pot components: effects on tomato plant growth and morphology, performance of an innovative two-stage process converting food waste to hydrogen and methane, soil organic matter properties after 40 years of different use of organic and mineral fertilisers, agricultural sustainability and intensive production practices, related papers.
Showing 1 through 3 of 0 Related Papers
- Open access
- Published: 14 January 2017
Comparative effectiveness of different composting methods on the stabilization, maturation and sanitization of municipal organic solid wastes and dried faecal sludge mixtures
- Tesfu Mengistu 1 ,
- Heluf Gebrekidan 1 ,
- Kibebew Kibret 1 ,
- Kebede Woldetsadik 2 ,
- Beneberu Shimelis 1 &
- Hiranmai Yadav 1
Environmental Systems Research volume 6 , Article number: 5 ( 2018 ) Cite this article
21k Accesses
39 Citations
1 Altmetric
Metrics details
Composting is one of the integrated waste management strategies used for the recycling of organic wastes into a useful product. Composting methods vary in duration of decomposition and potency of stability, maturity and sanitation. This study was aimed to investigate the comparative effectiveness of four different methods of composting viz. windrow composting (WC), Vermicomposting (VC), pit composting (PC) and combined windrow and vermicomposting (WVC) on the stabilization, maturation and sanitization of mixtures of municipal solid organic waste and dried faecal sludge.
The composting treatments were arranged in a completely randomized block design with three replications. The changes in physico-chemical and biological characteristics of the compost were examined at 20 days interval for 100 days using standard laboratory procedures. The analysis of variance was performed using SAS software and the significant differences were determined using Fisher’s LSD test at P ≤ 0.05 level.
The evolution of composting temperature, pH, EC, \({\text{NH}}_{ 4}^{ + }\) , \({\text{NO}}_{ 3}^{ - }\) , \({\text{NH}}_{ 4}^{ + }\) : \({\text{NO}}_{ 3}^{ - }\) ratio, OC, C:N ratio and total volatile solids varied significantly among the composting methods and with composting time. The evolution of total nitrogen and germination index also varied significantly (P ≤ 0.001) with time, but their variation among the composting methods was not significant (P > 0.05). Except for PC, all other methods of composting satisfied all the indices for stability/maturity of compost at the 60th day of sampling; whereas PC achieved the critical limit values for most of the indices at the 80th day. A highly significant differences (P ≤ 0.001) were noted among the composting methods with regard to their effectiveness in eliminating pathogens (faecal coliforms and helminth eggs). The WVC method was most efficient in eliminating the pathogens complying with WHO’s standard.
Turned windrow composting and composting involving earthworms hastened the biodegradation process of organic wastes and result in the production of stable compost earlier than the traditional pit method of composting. The WVC method is most efficient in keeping the pathogens below the threshold level. Thus, elimination of pathogens from composts being a critical consideration, this study would recommend this method for composting organic wastes involving human excreta.
As in many other cities of the developing countries, the rapid urbanization and high population growth of Dire Dawa (Ethiopia’s 2nd largest city) have resulted into a significant increase in generation of wastes from domestic and commercial activities, posing numerous questions concerning the adequacy of the current waste management systems, and their associated environmental, economical and social implications. A report by Beneberu et al. ( 2012 ) depicted that, despite the great efforts made by the Dire Dawa city municipality, it has been hardly possible to meet the ever-increasing waste management service demand of the city adequately and effectively. The per capita waste generation rate of the city is reported to be 0.3 kg day −1 and the city generates an estimated quantity of 77 tonnes of solid wastes per day (Community Development Research 2011 ). The same report indicated that, as there is very limited or no effort to recycle, reuse or recover the waste that is being generated; waste disposal has been the major mode of waste management practice. It has been observed that the indiscriminate dumping of wastes into the landfill is resulting in unexpectedly faster filling up of the city’s sanitary landfill which would, thus, likely be abandoned in the near future than anticipated 30 years (Beneberu et al. 2012 ).
In addition to the municipal solid wastes (MSW), the human excreta also constitute a significant component of wastes generated from Dire Dawa city. Faecal sludge (FS) accumulating in the commonly used on-site sanitation systems are periodically collected and dumped indiscriminately into its well-engineered sludge dewatering and drying bed. The faecal sludge, after being dried in the beds, since it has no purpose in Dire Dawa, was observed to be excavated from the drying beds and disposed in the landfill site. It is, therefore, of paramount importance to establish economically viable, environmentally sustainable and socially acceptable method of waste management for the sustainable development of the city.
Bundela et al. ( 2010 ) suggested that agricultural application of organic solid wastes, as nutrient source for plants and as soil conditioner, is the most cost effective municipal solid waste (MSW) disposal option because of its advantages over traditional means, such as land filling or incineration. Though, human wastes are a rich source of organic matter and inorganic plant nutrients and therefore used to support food production, their use without prior stabilization represents a high risk because of the potentially negative effects of any phytotoxic substances or pathogens they may contain (Garcia et al. 1993 ). Application of raw wastes may inhibit seed germination, reduce plant growth and damage crops by competing for oxygen or causing phytotoxicity to plants due to insufficient biodegradation of organic matter (Brewer and Sullivan 2003 ; Cooperband et al. 2003 ). Moreover, the reuse of untreated faeces for agricultural purposes can cause a great health risk, because a great number of pathogens such as bacteria, viruses and helminthes can be found in human excreta (Gallizzi 2003 ). Therefore, the management of urban solid wastes involving human excreta for recycling in agriculture should necessarily incorporate sanitization, stabilization and maturation aspects to minimize potential disease transmission and to obtain a more stabilized and matured product for application to soil (Carr et al. 1995 ).
Composting and vermicomposting are two of the best-known processes for biological stabilization of solid organic wastes by transforming them into a safer and more stabilized material that can be used as a source of nutrients and soil conditioner in agricultural applications (Lazcano et al. 2008 ; Bernal et al. 2009 ; Domínguez and Edwards 2010 ). Composting involves the accelerated degradation of organic matter by microorganisms under controlled conditions, in which the organic material undergoes a characteristic thermophilic stage that allows sanitization of the waste by elimination of pathogenic microorganisms (Lung et al. 2001 ). Vermicomposting, on the other hand, is emerging as the most appropriate alternative to conventional aerobic composting (Yadav et al. 2010 ) and it involves the bio-oxidation and stabilization of organic material by the joint action of earthworms and microorganisms (Lazcano et al. 2008 ). More recently, combining thermophilic composting and vermicomposting has been considered as a way of achieving stabilized substrates (Tognetti et al. 2007 ). Thermophilic composting results in sanitization of wastes and elimination of toxic compounds while the subsequent vermicomposting reduces particle size and increases nutrient availability (Mupondi et al. 2010 ).
Composting methods differ in duration of decomposition and potency of stability and maturity (Iqbal et al. 2012 ). Due to the ecological and health concerns of human wastes, extensive research has been conducted to study the composting process and to evaluate methods to describe the stability, maturity and sanitation of compost prior to its agricultural use (Brewer and Sullivan 2003 ; Zmora-Nahum et al. 2005 ). Although several studies have addressed the optimization of composting, vermicomposting or composting with subsequent vermicomposting of various organic wastes (Dominguez et al. 1997 ; Frederickson et al. 1997 ; Ndegwa and Thompson 2001 ; Tognetti et al. 2005 , 2007 ; Lazcano et al. 2008 ; Mupondi et al. 2010 ), information on the effectiveness of the different composting methods on biodegradation and sanitization of mixtures of MSW and dried faecal sludge (DFS) is scant. Moreover, regarding the sanitization efficiency of the different composting techniques, controversial reports have been presented in different literatures. Several researchers reported the effectiveness of thermophilic composting in eliminating pathogenic organisms (Koné et al. 2007 ; Vinnerås 2007 ; Mupondi et al. 2010 ). However, a few studies on composting of source-separated faeces claimed that a sufficiently high temperature for pathogen destruction is difficult to achieve (Bjorklund 2002 ; Niwagaba et al. 2009 ). Similarly, in vermicomposting, some studies have provided evidence of suppression of pathogens (Monroy et al. 2008 ; Rodriguez-Canche et al. 2010 ; Eastman et al. 2001 ), while others (Bowman et al. 2006 ; Hill et al. 2013 ) demonstrated the insignificant effect of vermicomposting in reducing Ascaris summ ova as compared to composting without worms. The effectiveness of vermicomposting for pathogen destruction was still remaining unclear due to conflicting information in the literature (Hill et al. 2013 ); the present scenario thus, calls for further exploration. Accordingly, the present study attempted to investigate the comparative effectiveness of four different methods of composting viz. windrow composting (WC), Vermicomposting (VC), pit composting (PC), and combined windrow and vermicomposting (WVC) on the stabilization, maturation and sanitization of mixtures of MSW and dried faecal sludge.
Experimental site, wastes and earthworms utilized
The study was carried out at Dire Dawa, a city in Eastern Ethiopia located at 9° 6′ N, 41° 8′ E and at an altitude of 1197 m above sea level. The Municipal solid organic waste used in this study was obtained from a door-to-door waste collection service provided by the Sanitation and Beautification Agency (SBA) of Dire Dawa city, in which the wastes were collected from various locations in the city. The dried faecal cake which was about to be excavated from the drying bed and dumped to the landfill site was collected from the dumping site. The garbage receives mixed organic and inorganic domestic wastes, upon arrival to the composting site; the wastes were spread flat on the ground and sorted manually into organic and non-organic fractions. All the compostable components were shredded manually into small pieces of particle sizes ranging from 3 to 5 cm as described by Pisa and Wuta ( 2013 ). The shredded MSW and dried faecal sludge were then mixed manually in a 2:1 mix ratio. The earthworm species ( Eisenia foetida ) were obtained from Haramaya University. Matured earthworms and their cocoons were brought to Dire Dawa, where they were made to be multiplied (reared) for about 4 months using cow dung as medium.
Composting treatments
The methods of composting tested were: turned windrow composting (WC), pit composting (PC) (a composting method commonly practiced by farmers of the study area), vermicomposting (VC) and combined windrow and vermicomposting (WVC). The composting was done in outdoor but under shade condition. Three replicates of each of the four composting methods were made being arranged in a completely randomized block design. Each composting pile was covered with a layer of dry grass (5 cm) to prevent excessive loss of moisture.
Windrow composting : In the thermophilic composting, the homogenized feedstock of 1 m 3 volume (~275 kg dry weight) was heaped into conical piles in about 1 m 2 area after being wetted with water to 50–60% (Maso and Blasi 2008 ).
Pit composting a homogenized feedstock with the same moisture level as in ‘a’ was filled in a pit with dimension of 1 × 1 × 1 m (length width and depth).
Vermicomposting : Vermicomposting was performed in vermicompost bed measuring 1 × 1 × 0.3 m (length, width and height respectively) framed with bricks where the walls and bottom of the structure was lined with polyethylene sheet. In order to drain the excess water, the bottom of the polyethylene sheet was made to have tiny holes. Mature earthworms ( E. foetida ) were introduced at the recommended stocking rate of 250 adult worms per 20 kg of bio-waste (Padmavathiamma et al. 2008 ). The moisture content of the material was maintained between 70 and 80% (Maso and Blasi 2008 ).
Combined windrow composting and vermicomposting : Thermophilic composting of the wastes was done in same manner as in windrow composting and the piled substrate was allowed to be composted until the temperature was dropped to mesophilic phase. After the completion of the thermophilic phase (15 days after the initiation of the process), the subsequent vermicomposting continued using earthworms ( E. foetida ) as described under vermicomposting (Mupondi et al. 2010 ) .
The pilled heaps in WC were turned and mixed every week while the substrates in other methods of composting were left intact. The moisture content of each pile was checked every week and adjusted accordingly. The compost mass in WVC received the same treatment as WC and VC during the thermophilic and mesophilic phases of composting respectively. The temperatures in each heap was measured daily with a temperature probe from randomly selected places (centre, bottom and top) throughout the process.
Compost sampling and analysis
Sampling procedure.
To evaluate the various physical, chemical and biological transformations of the compost, representative samples were collected from four different points of the compost pile (bottom, surface, side and centre) of each pile at every 20 days (20, 40, 60, 80 and 100 days). All the samples were sealed in plastic containers and transported immediately to the laboratory using an ice box. Up on their arrival to the laboratory, the samples were stored in a refrigerator at 4 °C until they were analysed. Physico-chemical and microbial analyses were carried out at Haramaya University following standard procedures.
Physico-chemical analysis of compost
Moisture content was determined as weight loss upon drying in an oven at 105 °C to a constant weight (Lazcano et al. 2008 ). Total nitrogen (TN) and organic carbon (OC) were determined using dried compost samples which were ground to pass through a 2-mm sieve as described by Pisa and Wuta ( 2013 ). For the determination of total N, samples were decomposed using concentrated H 2 SO 4 and catalyst mixture in Kjeldahl flask and subsequently, N content in the digest was determined following steam distillation and titration method (Bremner and Mulvaney 1982 ).Organic carbon was estimated by dichromate wet digestion and rapid titration methods as described by Walkley and Black ( 1934 ). Total volatile solids was determined as weight loss on ignition at 550 °C for 4 h in a muffle furnace as described by Lazcano et al. ( 2008 ). Ammonium N ( \({\text{NH}}_{ 4}^{ + }\) –N) was determined from 0.2 ml aliquot of 0.5 M K 2 SO 4 extract of the filtrate after colour development with sodium nitroprusside, whereas, Nitrate N ( \({\text{NO}}_{ 3}^{ - }\) –N) was determined in a separate aliquot (0.5 ml) after colour development with 5% salicylic acid using a spectrophotometer (Okalebo et al. 2002 ). Analysis for pH and electrical conductivity (EC) were performed in extracts of 1:10 (w/v) compost: distilled water ratio as described by Ndegwa and Thompson ( 2001 ). The C:N ratio was calculated using the individual values of OC and TN.
Compost phytotoxicity test
For determining compost phytotoxicity, a modified phytotoxicity test employing seed germination was used (Zucconi et al. 1981 ). A 10 g of screened compost sample was shaken with 100 ml of distilled water for an hour, then the suspension was centrifuged at 3000 rpm for 15 min and the supernatant was filtered through a Whatman No 42 filter paper. Number 2 Whatman filter paper was placed inside a sterilized petri dish and wetted with 9 ml of the extract, 30 tomato seeds ( Solanum esculentum L.) were placed on the paper. Nine ml of distilled water was used as a control and all experiments were run in triplicate (Wu et al. 2000 ). The petri dishes were kept in the dark for 4 days at room temperature. At the end of the 4th day, the germination index (GI) was calculated using the following formula (Selim et al. 2012 ).
Faecal coliform analysis
For the determination of faecal coliforms in the initial raw materials and in the composts the procedures described by Mupondi et al. ( 2010 ) were employed. Aseptically weighed 10 g samples of either waste mixture or fresh compost were added to 90 ml of distilled water previously autoclaved at 121 °C for 15 min and the suspensions were then mixed using a blender to ensure thorough mixing. Additional serial dilutions were made up to 10 −6 . A 0.1 ml aliquot of each dilution was plated, in triplicate, in appropriate media-Violet Red Bile Agar (VBA) (Vuorinen and Saharinen 1997 ). The plates were then maintained in an incubator at a constant temperature of 44 °C for 24 h. For each of the treatment samples the numbers of faecal coliforms were expressed as log 10 CFU (colony forming unit) per gram of fresh sample and average values were calculated.
Helminth eggs recovery
The determination of helminth egg in this study was done based on the US EPA protocol ( 1999 ) modified by Schwartzbrod ( 2003 ). The analysis was carried out in triplicate for the initial raw waste and compost samples. The concentration of number of eggs per gram of dry weight of sample was computed according to the following formula (Ayres and Mara 1996 ):
where N = number of eggs per gram of dry weight of sample, Y = number of eggs in the McMaster slide (mean of counts from three slides), M = estimated volume of product at final centrifugation, C = volume of the McMaster slide, S = dry weight of the original sample.
Data analysis
The data obtained from this study were subjected to statistical analysis of variance (ANOVA) procedures using SAS software and the significant differences were determined using Fisher’s LSD test at P ≤ 0.05 level.
Results and discussion
Characteristics of the raw waste materials.
The results of the analysis for the raw wastes are presented in Table 1 . The pH of the municipal solid waste (MSW) was alkaline and that of dried faecal sludge (DFS) was acidic in reaction. EC of MSW was much greater than that of DFS. The alkaline pH and high EC value in MSW could be attributed to the presence of wood ash which was observed to occur in considerable amount during the screening of the waste. The total N content of DFS was more double than that of MSW, indicating that it could be used to reduce the C:N ratio of the MSW.
The total helminth egg count for the dried faecal sludge and mixture of faecal sludge and MSW was 80.56 g −1 TS and 38.89 g −1 TS respectively, which is far greater than the recommended value for materials used in agriculture as per WHO’s guidelines (≤3–8 eggs g −1 TS) (Xanthoulis and Strauss 1991 ). Similarly, the total faecal coliform count of all the raw materials was found to exceed the standard threshold limit of <1000 cfu g −1 (WHO 2006 ). Therefore, it suggests that the raw wastes cannot be used directly for agriculture without being treated as it may result in soil contamination. The germination index values of the wastes was also far below the standard limit (>80%) substantiating the presence of phytotoxic substances which would make the raw wastes unfit for application in agricultural soils (Additional file 1 : Table S1).
Evolution of composting temperature
Considerable variations in temperature conditions were observed among the different composting methods on course of the composting period (Fig. 1 ). Though there were series of rise and fall in temperature, the general pattern of temperature for treatments (particularly for WC and PC) was similar. There was a rapid rise in temperature during the first few days of the composting process followed by a fall with time and finally it began to gradually reach to the ambient temperature. These temperature patterns denoted the thermophilic, mesophilic and maturation phases of a composting process, respectively. The rapid progress from initial mesophilic phase to thermophilic phase in WC and PC indicates a high proportion of readily degradable substances and self-insulating capacity of the waste (Sundberg et al. 2004 ). The change in temperature pattern observed in this study is in accord with other composting study (Tognetti et al. 2007 ).
Changes in ambient air temperature and temperature in the experimental piles during the composting process ( WC windrow composting, VC vermicomposting, PC pit composting, WVC combined windrow and vermicomposting)
Temperatures reached the thermophilic range (>45 °C) on the second and third day for the WC and PC which lasted for 15 and 19 days, respectively after initiation of the process. During these days of the process, a higher temperature was recorded for the WC than the PC. A peak average temperature ranging between 60.7 and 62.67 °C was recorded during the 3rd to 6th days for WC. Correspondingly for PC, the highest average temperature of 50.2–52.4 °C was registered during the 3rd to 9th day (Additional file 1 ). The increase in temperature within the composting mass was caused when the heat generated from the respiration and decomposition of sugar, starch and protein by the population of microorganisms accumulates faster than it is dissipated to the surrounding environment (Jusoh et al. 2013 ).
During the subsequent mesophilic phase (45–35 °C), however, PC registered a relatively higher temperature than WC. This phase was lasted for 13 days, from 16th to 28th day for WC and from 20th to 32nd day for PC and from the respective days on temperature values <35 °C and very close to the ambient temperature was recorded for both composting methods. The ambient temperature during the experimental period ranged from 23.7 to 33.7 °C (Fig. 1 ).
The vermicomposting unit (VC), where low temperature was induced intentionally by spreading the material in ground beds, tended to show the lowest temperature all through the process. The temperature profile for the WVC during the thermophilic phase showed similar pattern as that of the WC and has taken a different track during the subsequent vermicomposting process resembling the sole vermicomposting unit.
The size, initial moisture content and aeration of the piled substrate might have attributed for the variation in temperature of the different composting methods. Initially, to protect the earthworms from extreme thermophilic temperature and to keep an optimum condition for their performance, the height and moisture content of the pile in the vermicomposting unit were maintained to 30 cm and 80% compared to 1 m height/depth and 60%, respectively, in the WC and PC piles. As a result, the vermicompost with small volume of organic pile and relatively high moisture content does not heat up as such because the heat generated by the microbial population is lost quickly to the atmosphere, whereas in the WC and PC heat build-up particularly in the centre of the pile might have been insulated by the outer layer letting the temperature inside the pile to be raised. It is a well-established fact that, the smaller the bioreactor or compost pile, the greater the surface area-to-volume ratio, and therefore the larger the degree of heat loss to conduction and radiation ( http://www.cfe.cornell.edu/compost/invertebrates.html ).
The possible explanation for the variation in temperature profile of the WC and PC, given the same volume and moisture content of the pile, may be the differences in aeration (air circulation) in the piled substrates. The weekly turning of the compost mass in WC might have promoted the free circulation of air to enhance the microbial activity in the oxidation process and thereby raise the temperature; whereas in PC, the substrates being stacked in the pit without being turned the circulation of air in the pile might have been relatively restricted to impair the microbial activity and thereby the heat generated during the process. Finstein et al. ( 1986 ) who demonstrated the linear relationship between the oxygen consumed and heat produced during aerobic metabolism, support the finding of this study.
Evolution of pH
The first pH reading being taken at the 20th day after the initiation of the process, a sharp and significant (P ≤ 0.001) rise in pH than the initial state was observed in all the treatments. The rise in pH during these days is considered to be the result of the metabolic degradation of organic matter containing nitrogen (proteins, amino acids etc.) leading to formation of amines and ammonia salts through mineralization of organic nitrogen (Dumitrescu et al. 2009 ). As Smith and Hughes ( 2002 ) and Mupondi et al. ( 2006 ) suggested, it might also be attributed to the decomposition of organic acids to release alkali and alkali earth cations previously bound by organic matter. An increase in pH during composting of different substrates was also reported in many other studies (Sundberg et al. 2004 ; Tognetti et al. 2007 ; Gao et al. 2010 ).
The analysis of variance (ANOVA) showed a non-significant variation (P > 0.05) of pH values among the different methods of composting at the 20th day of sampling. Nevertheless, as composting progressed, significant variation (P ≤ 0.01) in pH was noted among the different composting methods (Fig. 2 ). Except for PC, which exhibited a further rise in pH, all other methods of composting showed a fairly stable pH during the 20th to 60th day of the process. This was followed by a slight fall to nearly neutral pH value during 80th to 100th day. In PC, a rise in pH value was observed to extend to the 60th day (8.03), after which it declined slightly at the 80th day and finally dropped to 7.83 at the 100th day.
Changes in pH in different composting methods with time. ( WC windrow composting, VC vermicomposting, PC pit composting, WVC combined windrow and vermicomposting, LSD least significant difference). Different letters indicate significant differences at P ≤ 0.05
Generally, from the 20th day till the end of the process (100th day), PC registered the highest pH value than the rest of the composting methods which were noted for their statistical parity (P > 0.05) (Fig. 2 ). This may possibly be caused due to the relatively higher concentration of ammonium ion maintained in PC. The relative decline in pH during the latter stage of the composting process might be caused due to the nitrification process which is responsible for the release of H + ion (Huang et al. 2001 ). This is also evident from \({\text{NO}}_{ 3}^{ - }\) data which was observed to increase remarkably during later stages of the process. Overall, the pH values achieved in all treatments at the end of the experiment were within the range acceptable for plant growth as recommended by Tognetti et al. ( 2005 ).
Evolution of electrical conductivity (EC)
The electrical conductivity values varied significantly (P ≤ 0.01) among the composting methods and over the different composting period. Generally, as indicated in Fig. 3 , all the treatments showed similar pattern of change in EC where the value decreased steadily with the progress in the composting process. It was found to be reduced by about 55.53, 54.66, 47.97, and 37.40% respectively for VC, WVC, PC, and WC at the 100th day as compared to the initial value of the raw material at day 0. The obtained results are in agreement with Yadav et al. ( 2012 ) and Gao et al. ( 2010 ) who reported an eventual decrease in EC value with progress in composting and vermicomposting. However this is in contrast with other studies (Gómez-Brandón et al. 2008 ) which reported increased EC values with composting time.
Changes in EC in composting mixtures of different composting methods with time. ( WC windrow composting, VC vermicomposting, PC pit composting, WVC combined windrow and vermicomposting). Different letters indicate significant differences at P ≤ 0.05
The progressive decline of EC value with time would justify that, firstly; there might be leaching of mineralized ions during periodic showering of water on the composting mass, secondly; as composting process progressed, humification would inevitably proceed and the resulting humic fractions might have complexed the soluble salts which in turn tend to decrease the amount of mobile free ions and thereby the EC (Rao 2007 ).
The ANOVA results revealed that the EC value during the entire composting period was significantly higher (P ≤ 0.001) for WC followed by PC, whereas VC which was in statistical parity with WVC recorded the lowest value (Fig. 3 ). This would justify that the piled substrates in PC, VC and WVC which were not turned, but rather watered periodically on top to maintain the moisture at optimum; the soluble ions might have gradually been leached down. Moreover in VC and WVC, owing to the smaller size of the pile and a relatively large quantity of water added, the leaching of those ions might have been even more pronounced than the PC. In WC on the other hand, the weekly turning and mixing up of the substrate might have helped the redistribution of the mineralized ions in the compost mass and hence the loss of those ions from the system through leaching might have relatively been reduced. This finding is in line with Lazcano et al. ( 2008 ) and Frederickson et al. ( 2007 ) who reported a significantly lower EC value for VC and WVC than WC. The EC value in the final product of all treatments was far below the threshold value of 3000 µS cm −1 indicating a material which can be safely applied to soil (Soumaré et al. 2002 ).
Evolution of total organic carbon
With advancement of the composting process, the total organic carbon content of the compost decreased consistently and significantly (P ≤ 0.01) for all the treatments (Fig. 4 ). The decrease in organic carbon content at the end of the composting process with respect to WVC, VC, WC and PC was 54.74, 54.52, 52.00, and 48.80%, respectively of their initial carbon content. The present finding is also in consent with the findings of Tiquia et al. ( 2002 ), who reported a total carbon loss that ranged from 50 to 63% in turned windrows and 30–54% in unturned windrows. Similarly, reviewing the works of other authors, Yadav et al. ( 2010 ) reported total organic carbon reduction values ranging between 26 and 66% during vermicomposting of wastes of various sources. The variation in the amount of OC lost from the different composting method may possibly be caused by differences in the aeration of the piled substrate. Turning the compost pile (in WC) and continuous borrowing and fragmenting of the material by earthworms (in VC and WVC) might have altered the aeration of the compost mass and accelerated the degradation process to enhance the loss of carbon as carbon dioxide. The results are in agreement with the findings of Guo et al. ( 2012 ) who demonstrated higher losses of carbon in treatments receiving higher rates of aeration.
Changes in total organic carbon in composting mixture of different composting methods with time. ( WC windrow composting, VC vermicomposting, PC pit composting, WVC combined windrow and vermicomposting). Different letters indicate significant differences at P ≤ 0.05
Evolution of total nitrogen
Changes in the total nitrogen of the different composting methods varied significantly (P ≤ 0.01) with the different sampling period, while the variation among the composting methods was found to be statistically insignificant (P > 0.05) (Fig. 5 ). The total nitrogen content of the initial raw material of all treatments was reduced significantly (P ≤ 0.01) during the first 20 days of composting. However, during the subsequent sampling, there was a gradual increment of total nitrogen, the maximum value being recorded at the 100th day. The decline in the total nitrogen during the first 20 days might be attributed to the loss of nitrogen in the form of ammonia which is apparent during the active phase of composting. Witter and Lopez-Real ( 1988 ) reported nitrogen losses that could amount to 50% and considered that nearly all nitrogen lost is due to ammonia volatilization.
Changes in total nitrogen in composting mixture of different composting methods with time ( WC windrow composting, VC vermicomposting, PC pit composting, WVC combined windrow and vermicomposting). Different letters indicate significant differences at P ≤ 0.05
The rise in total nitrogen after the 20th day may be caused due to a concentration effect that resulted from degradation of organic C compounds which in turn leads to weight loss and therefore, a relative increase of N concentration (Dias et al. 2010 ). As Bernal et al. ( 1998 ) explained the concentration of N usually increases during composting when the loss of volatile solid (organic matter) is greater than the loss of NH 3 . This would generally indicate that there was a relatively greater increase in total N compared with the decrease in the organic carbon content. The results of the present study would, therefore, justify that during the first 20 days of composting, losses of N through NH 3 volatilization occurred at a greater rate than organic matter degradation, while during the subsequent periods, the rate of N loss as NH 3 might be slower than the rate of dry matter loss as CO 2 . In addition, the N level might have also been increased due to the fixation of atmospheric N within the compost heap by the free living N fixing microorganisms’ activity that commonly occurs during the later stage of the composting process (Seal et al. 2012 ). In their co-composting study of pig manure and corn stalks, Guo et al. ( 2012 ) reported results that were in agreement with the trends of the present study—a general decrease of total nitrogen during the thermophilic phase followed by an increase then after.
Evolution of C:N Ratio
The C:N ratio of the composting material of all the treatments narrowed consistently and significantly (P ≤ 0.01) with the advancement of the composting time (Fig. 6 ). The initial C:N ratio of the raw material at day 0 was 19:1 which was within the recommended range suitable for composting (35–12) (Epstein 1997 ). This was found to decrease to nearly 11:1, 9:1, 10:1 and 9:1 at the 100th day of sampling for PC, VC, WC and WVC, respectively. Obviously, throughout the composting process the organic matter is decomposed by microorganisms through which the organic carbon was oxidized to CO 2 gas to the atmosphere and thus lowers the C:N ratio (Jusoh et al. 2013 ). This is in conformity with the findings of other studies (Kumar et al. 2009 ; Khwairakpam and Kalamdhad 2011 ).
Changes in C:N ratio of composting mixture in different composting methods with time ( WC windrow composting, VC vermicomposting, PC pit composting, WVC combined windrow and vermicomposting). Different letters indicate significant differences at P ≤ 0.05
C:N ratio value for PC was significantly (P ≤ 0.01) higher than the other methods of composting which were statistically at par (P > 0.05) with each other (Fig. 6 ). The variation seemed to arise mainly due to the differences in the amount of total organic carbon as could be witnessed from previous discussion and the same justification given above can also be claimed for the variation in C:N ratio among the different composting methods. Generally, the C:N ratios in the final product of all the treatments were found to be satisfactory because matured compost material usually has a C:N ratio of 15 or less (Hock et al. 2009 ).
As Gómez-Brandón et al. ( 2008 ) pointed out C:N ratio may not be a good indicator of compost stability because it can level off before the compost stabilizes. When wastes rich in nitrogen are used as source material for composting, the C:N ratio can be within the values of stable compost even though it may still be unstable. By the same token, Zmora-Nahum et al. ( 2005 ) reported a C:N ratio lower than the cut-off value of 15 very early during the composting of cattle manure, while important stabilization processes were still taking place. Correspondingly, in the present study, three of the four treatments (VC, WVC and WC) and PC achieved a C:N ratio of <15 at the 40th and 60th day of sampling, respectively, while the degradation of the organic material was still significant till the 60th and 80th days for the respective treatments. As evidenced earlier a statistically stable values for total organic carbon was observed during the 60th to 100th and 80th to 100th day of sampling for the respective treatments.
Evolution of \({\text{NH}}_{ 4}^{ + }\) , \({\text{NO}}_{ 3}^{ - }\) and \({\text{NH}}_{ 4}^{ + }\) :NO 3 ratio
The concentration of \({\text{NO}}_{ 3}^{ - }\) –N and \({\text{NH}}_{ 4}^{ + }\) –N varied significantly (P ≤ 0.001) for the different composting methods and over the different composting period, notwithstanding that all the treatments have generally shown similar pattern of changes in both ammonium and nitrate concentrations (Figs. 7 , 8 ). As can be seen from the graph (Fig. 7 ), all the composting methods showed a rise in \({\text{NH}}_{ 4}^{ + }\) –N concentration during the 20th day of sampling which was then declined sharply as evidenced at the 40th day and coming to decrease slightly from the 40th day until the end of the experiment (100th day).
Changes in \({\text{NH}}_{ 4}^{ + }\) concentration of composting mixture in different composting methods with time ( WC windrow composting, VC vermicomposting, PC pit composting, WVC combined windrow and vermicomposting). Different letters indicate significant differences at P ≤ 0.05
Changes in \({\text{NO}}_{ 3}^{ - }\) concentration of composting mixture in different composting methods with time ( WC windrow composting, VC vermicomposting, PC pit composting, WVC combined windrow and vermicomposting). Different letters indicate significant differences at P ≤ 0.05
The rise in \({\text{NH}}_{ 4}^{ + }\) –N concentration during the first 20 days was likely to be caused as a result of the mineralization of organic matter (the conversion of organic N to \({\text{NH}}_{ 4}^{ + }\) via the ammonification process), thus reflecting active transformation of organic matter and unstable substrate (Tognetti et al. 2005 ; Guo et al. 2012 ). Whereas the decrease in \({\text{NH}}_{ 4}^{ + }\) –N during the subsequent sampling periods was probably due to NH 3 volatilization (Gao et al. 2010 ), the microbial immobilization as nitrogenous compounds such as amino acids, nucleic acids and proteins and/or its oxidation to \({\text{NO}}_{ 3}^{ - }\) through nitrification process (Guo et al. 2012 ). An increase in \({\text{NH}}_{ 4}^{ + }\) –N concentration during the initial stage of composting and its reduction afterwards was reported by Gao et al. ( 2010 ).
The analysis of variance indicated that PC registered the highest concentration of \({\text{NH}}_{ 4}^{ + }\) –N during all the sampling period. However, a statistically significant (P ≤ 0.01) variation of \({\text{NH}}_{ 4}^{ + }\) –N among the treatments was recorded only at the 20th and 40th day of sampling (Fig. 7 ). Turning the piled substrate in WC and the smaller size and increased surface area of the vermibed in VC and WVC might have resulted in increased loss of ammonia leading to a relatively low level of ammonium at this day of sampling (20th day). The compost pile in PC, on the other hand, being not turned and mixed, the loss of N in the form of ammonia might have relatively been reduced and this might have contributed for the increased level of ammonium nitrogen in PC than the other methods of composting. Similar results were reported by Guo et al. ( 2012 ) who noted highest level of ammonium nitrogen in treatments with low than high aeration rate.
Regarding the \({\text{NO}}_{ 3}^{ - }\) –N, for all the treatments its level was sharply and significantly (P ≤ 0.01) decreased at the 20th day sampling than the initial. This might be caused due to either the leaching of nitrate by water during periodic watering of the composting mass or its immobilization by the decomposing microorganisms. During the subsequent composting period (20th to 60th days), however, the \({\text{NO}}_{ 3}^{ - }\) –N level came to be relatively stable and during these days the variation in \({\text{NO}}_{ 3}^{ - }\) –N level among all the treatments was insignificant (P > 0.05) (Fig. 8 ). This was followed by a sharp rise of \({\text{NO}}_{ 3}^{ - }\) –N after the 60th day (for WC, VC and WVC) and 80th day (for PC) as evidenced on the 80th and 100th day of sampling, respectively. At the end of the process (100th day), PC exhibited a significantly lower value of \({\text{NO}}_{ 3}^{ - }\) –N than the other methods of composting. It seems that due to the better aeration by earthworms (in VC and WVC) and turning of the piles (in WC), the oxidation of \({\text{NH}}_{ 4}^{ + }\) to \({\text{NO}}_{ 3}^{ - }\) might have been enhanced in the respective methods of composting than in PC.
The \({\text{NH}}_{ 4}^{ + }\) –N content of the starting material was clearly higher (1014.28 mg kg −1 ) than the \({\text{NO}}_{ 3}^{ - }\) –N content (684.5 mg kg −1 ), giving the \({\text{NH}}_{ 4}^{ + }\) : \({\text{NO}}_{ 3}^{ - }\) ratio to be 1.48. On course of the composting process the ratio was found to be raised sharply at the 20th day of sampling for all the treatments. This is followed by a drastic decline during the 40th day and coming to be declining gradually during the subsequent periods of composting (60–100 days) (Fig. 9 ). PC registered the highest ratio during all the sampling periods; however, a statistically significant variation among the composting treatments was noted only at the 20th and 40th day of sampling (Fig. 9 ). At the 20th day, the highest (13.57) and lowest (9.42) ratio was recorded for PC and WVC, respectively. At the 100th day of sampling the value was found to drop to 0.06, 0.026, 0.016 and 0.02, respectively for PC, VC, WC and WVC.
Changes in \({\text{NH}}_{ 4}^{ + }\) : \({\text{NO}}_{ 3}^{ - }\) ratio of composting mixture in different composting methods with time ( WC windrow composting, VC vermicomposting, PC pit composting, WVC combined windrow and vermicomposting). Different letters indicate significant differences at P ≤ 0.05
Critical limit values of <400 mg kg −1 for \({\text{NH}}_{ 4}^{ + }\) –N (Zucconi and de Bertoldi 1987 ), >300 mg kg −1 for \({\text{NO}}_{ 3}^{ - }\) –N (Forster et al. 1993 ) and <1 for \({\text{NH}}_{ 4}^{ + }\) -: \({\text{NO}}_{ 3}^{ - }\) ratio (Brewer and Sullivan 2003 ) has been established as a stability/maturity indices for composts of various origins. Concomitantly, except for PC all the other composting treatments satisfied the critical limits for stability/maturity at the 60th day of sampling. Whereas, PC achieved these values( \({\text{NO}}_{ 3}^{ - }\) –N and \({\text{NH}}_{ 4}^{ + }\) : \({\text{NO}}_{ 3}^{ - }\) ratio) at the 80th day, implying that PC was late to achieve the index value for maturity than the other three methods of composting and the same explanation given above pertaining to differences in aeration would also be suggested for the variation in these values among the treatments.
Evolution of total volatile solids (TVS)
The average total volatile solid (TVS) content of the raw waste was 523.4 mg kg −1 which steadily decomposed throughout the experimental period. The change in TVS with composting time showed the same pattern as the change in total organic carbon in that it decreases significantly (P ≤ 0.01) with the advancement of composting time. The greatest reduction in TVS was noted during the first 20 days of composting signifying the fast degradation of the substrate during this active phase of composting (Fig. 10 ). The decrease in TVS content of the sample indicates the degradation of organic matter of the waste during the composting process (Levanon and Pluda 2002 ). Values of TVS varied significantly (P ≤ 0.01) among the different methods of composting (Fig. 10 ). On the course of composting, the highest and lowest values of TVS were recorded for PC and WVC, respectively.
Changes in total volatile solids of composting mixture in different composting methods with time ( WC windrow composting, VC vermicomposting, PC pit composting, WVC combined windrow and vermicomposting). Different letters indicate significant differences at P ≤ 0.05
The analysis of variance revealed that the values of TVS for the three methods of composting (WC, VC and WVC) after the 60th day was insignificant (P > 0.05) indicating the stability of the product at the 60th day. Whereas, for PC a statistically stable value was achieved at the 80th day of composting, implying the relatively longer period of time the latter has taken for the product to be stable. This is due to the relatively slow rate of degradation of the organic matter in PC. The important role played by the earthworms in reducing the TVS through degrading wastes was reported by Yadav et al. ( 2012 ).
Phytotoxicity assessment
All the composting treatments followed the same general pattern of changes in germination index (GI) over the different sampling period and the variation in GI values among the treatments was insignificant (P > 0.05; Fig. 11 ). However, the values varied significantly (P ≤ 0.01) with the composting time. The lowest value of this variable was recorded at the 20th day of sampling which was of course statistically not different from the starting material (day 0). This was observed to increase with the advancement of composting period up to the 60th day and from the 60th day on it came to a more or less stable value with insignificant variation (Fig. 11 ). Tiquia and Tam ( 1998 ) also reported findings that are similar to the results of this study.
Changes in germination index (GI) of composting mixture in different composting methods with time ( WC windrow composting, VC vermicomposting, PC pit composting, WVC combined windrow and vermicomposting). Different letters indicate significant differences at P ≤ 0.05
The reason for the low germination index value of in the initial sample and the sample taken at the 20th day of the composting process could be attributed to the presence of phytotoxic compounds in the raw wastes and their production in the substrate during the active phase of composting. Phytotoxic compounds, such as; ammonium ions, fatty acids, and low molecular weight phenolic acids are reported to impair seed germination and root elongation (Delgado 2010 ; Gómez-Brandón et al. 2008 ). It was also evident from the chemical analysis of the raw material and compost samples of this study that the highest level of ammonium was recorded at the 20th day of sampling followed by the initial substrate at day 0. The detrimental effect of high levels of ammonium to seed germination and root elongation was reported in many other studies (Tiquia and Tam 1998 ; Selim et al. 2012 and Guo et al. 2012 ).
The rise in GI late at the 60th day might be due to the degradation of the phytotoxic compounds which were present in the initial raw wastes or produced during the active phase of composting as intermediate products of microbial metabolism (Bernal et al. 1998 ). According to Haq et al. ( 2014 ) compost with GI of more than 80% is considered to be matured and practically free of phytotoxic substances. In this study as indicated in the graph (Fig. 11 ), all the treatments were found to have a GI value of >80% at the 60th day of sampling, implying that, about 60 days were needed to overcome the threshold limit of 80% by reducing the phytotoxicity of the compost to levels consistent for a safe soil application (Soares et al. 2013 ).
Pathogen inactivation
Total faecal coliforms.
Except for VC all other methods of composting showed a substantial reduction in population of faecal coliforms at the 20th day of sampling. These treatments were effective in keeping the population of the faecal coliforms in the compost below the minimum allowable limit (<1000 cfu g −1 ) right at the 20th day. The reduction in the population of faecal coliforms in these methods of composting might be related to the high temperature generated in the compost pile during the thermophilic phase. Perhaps in this study the first sampling was taken at the 20th day, but it is likely that these methods could have attained such low population even much earlier than the 20th day. As per the reports of WHO ( 2006 ) and Schönning and Stenström ( 2004 ), pathogen inactivation in composting is achieved when temperatures above 50 °C are maintained for at least 1 week. Temperatures exceeding 50 °C were also recorded in those methods (WC, PC and WVC) involving thermophilic phase of the current study.
Some inconsistencies in reduction pattern of the faecal coliforms were detected in WC during the mesophilic and curing phase, where the population of these pathogens came to rise and fall at different sampling periods (Fig. 12 ). This may be due to the contamination of the compost mass from the external source during the periodic and manual turning of the compost pile.
Elimination of faecal coliform during co-composting of dried faecal sludge and municipal solid organic wastes with time. ( WC windrow composting, VC vermicomposting, PC pit composting, WVC combined windrow and vermicomposting). Different letters indicate significant differences at P ≤ 0.05
Regarding VC, contrary to the former methods, the number of the faecal coliforms was found to increase remarkably at the 20th day of sampling, this was then declined steadily during the subsequent sampling periods (Fig. 12 ). The increasing of faecal coliforms in VC during the 20th day of sampling could be attributed to creation of a good environment for multiplication of this pathogen through rehydration and subsequent availability of easily degradable substrates by dissolution following rehydration (Mupondi et al. 2010 ). The reports by Schönning and Stenström ( 2004 ) and WHO ( 2006 ) also indicated that certain types of pathogenic bacteria can increase in numbers when conditions favouring their growth are established in their storage medium/environment.
The reduction of the faecal coliforms population during the subsequent period of vermicomposting may be attributed to some activities of earthworms which possibly include: selective predation/consumption (Edward and Bohlen 1996 ; Kumar and Shweta 2011 ); mechanical destruction through action of gizzard (Edwards and Subler 2011 ); microbial inhibition through humic and coelomic acids or other enzymes secreted within the digestive tract (Edwards and Subler 2011 ); stimulation of microbial antagonists (Kumar and Shweta 2011 ); and indirectly through stimulation of endemic or other microbial species which outcompete, antagonize, or otherwise destroy pathogens (Edwards and Subler 2011 ).
Helminth egg count
During the composting process, there was a general reduction in the number of helminth eggs for all the treatments (Fig. 13 ). The total helminth egg count was found to decrease from 38.89 g −1 TS of the starting material to 8.33 (WC), 19.44(VC), 14.81 (PC) and 2.78 (WVC) in the final product as evidenced at the 100th day. These values correspond to a 78.57, 50, 61.9 and 92.86% total reduction of eggs for the respective treatments. It has been observed that the extent to which the helminth eggs were eliminated varied significantly with time and among the treatments (P ≤ 0.01). Those treatments involving thermophilic composting (WC, PC and WVC) demonstrated a drastic reduction of eggs during the first 20 days of the process when the active thermophilic phase was prevailing. This amounts to 84.85% (WC), 73.08% (PC) and 74.36% (WVC) of the total reductions recorded in the respective treatments. Whereas the treatment without a thermophilic phases (VC), the greatest reduction of helminth eggs was observed during the latter stages of the composting process. More than 75% of the total reduction was recorded after the 60th day of the process while only 23.81% of it was recorded during the first 40 days of the composting process.
Helminth eggs removal dynamics during co-composting of faecal sludge and municipal organic solid waste. ( WC windrow composting, VC vermicomposting, PC pit composting, WVC combined windrow and vermicomposting LSD Least significant difference). Different letters indicate significant differences at P ≤ 0.05
The highest reduction of eggs was achieved in WVC method followed by windrow method of composting (WC), while the sole vermicomposting method (VC) registered the lowest value (Fig. 13 ). However, only the former treatment (WVC) is complying with the WHO guidelines of <3–8 Ascaris egg g −1 TS while all the rest treatments were found to have egg counts more than the threshold limit. The result of this study clearly demonstrated that the high temperature produced in the thermophilic phase of the composting process is much more effective in sanitizing pathogenic parasites of faecal sludge than the earthworms did. It has been suggested that high temperature may increase the permeability of the Ascaris eggs’ shell, allowing transport of harmful compounds, as well as increasing the desiccation rate of the eggs (Koné et al. 2010 ).
Even though numerous authors reported the full elimination of parasitic eggs under thermophilic condition (Plym-Forshell 1995 ; Gantzer et al. 2001 ), this had not come about in the present study where helminth eggs were still detected despite the fact that the thermophilic condition (≥45 °C) was maintained for about 15–19 days. It is likely that the lethal temperature, being not evenly distributed throughout the piled biomass, the complete destruction of the eggs may not be ensured. The substrates that lay on the top of the pile, being exposed to the open atmosphere, might have experienced a relatively cooler temperature than the inner laid ones. Strauch ( 1991 ) suggested that composting ensures hygienization of the material on condition that all biomass is exposed to a sufficiently high temperature (55 °C for 14 days).
The temperature reading of the present study indicates that, on average, a high temperature of (>55 °C) was recorded only for 8 days in windrows and during which the pile was turned only once letting it to experience the high temperature of >55 °C for only a day after this first turning. This would therefore suggest that, had the piled feedstock been turned more frequently such that every 2 or 3 days, the biomass would have enjoyed the lethal high temperature uniformly and for relatively longer period of time and thus would have resulted in increased efficiency of helminth egg elimination. This justification is of course in argument with the reports of Koné et al. ( 2007 ) who demonstrated the non-significant effect of turning frequency on the inactivation efficiency of helminths egg. However, it has been explained that the size of the piled feedstock determines the magnitude of heat generated and the time duration in which the thermophilic phase would be maintained during the composting process. The larger the size of the pile the higher the magnitude of heat generated and the longer the thermophilic phase would be maintained within the pile, and thus the less frequently it can be turned. In cases where the pile size is smaller, the thermophilic phase would last for a short period of time; therefore, unless turned frequently there would be no chance for the out laid biomass to enjoy the lethal high temperature which is usually formed inside the pile. In the United States of America, the compost is regarded as hygienically safe if a temperature >55 °C is maintained in windrows for at least 15 days with a minimum of 5 turnings during the high temperature period (USEPA 1999 ).
Conclusions
The biodegradation process of organic wastes is markedly influenced by the methods of composting employed. Turned windrows (WC) and composting involving earthworms (VC and WVC) hasten the biodegradation process of organic wastes and result in the production of stable compost earlier than the traditional pit method of composting (PC). Even though all the tested methods of composting remarkably reduced the pathogenic organisms (faecal coliforms and helminth eggs), it was only the WVC method that qualify the standard set by WHO, keeping the concentration of helminth egg below the threshold level. Thus, elimination of pathogens from composts being a critical consideration, this study would recommend the WVC method for composting organic wastes involving human excreta.
Abbreviations
analysis of variance
colony forming unit
dried faecal sludge
electrical conductivity
germination index
faecal sludge
municipal solid waste
organic carbon
pit composting
total:nitrogen
total volatile solids
United States Environmental Protection Agency
vermicomposting
windrow composting
windrow plus vermicomposting
world health organization
Ayres RM, Mara DD (1996) Analysis of wastewater for use in agriculture—a laboratory manual of parasitological and bacteriological techniques. World Health Organization (WHO), Geneva
Beneberu S, Eline B, Harole Y, Zelalem L (2012) Current solid waste management practices for productive reuse in Dire Dawa City, Ethiopia (Draft project Report)
Bernal MP, Paredes C, Sanchez-Monedero MA, Cegarra J (1998) Maturity and stability parameters of composts prepared with a wide range of organic wastes. Bioresour Technol 63:91–99
Article CAS Google Scholar
Bernal MP, Alburquerque JA, Moral R (2009) Composting of animal manures and chemical criteria for compost maturity assessment: a review. Bioresour Technol 100:5444–5453
Bjorklund A (2002) The potential of using thermal composting for disinfection of separately collected faeces in Cuernacava, Mexico. Minor Field Studies No. 200. Swedish University of Agricultural Sciences, International Office. ISSN 1402-3237
Bowman DD, Liotta JL, McIntosh M, Lucio-Forster A (2006) Ascaris suum egg inactivation and destruction by the vermicomposting worm, Eisenia foetida . Residuals Biosolids Manag 2:11–18
Google Scholar
Bremner JM, Mulvaney CS (1982) Nitrogen—total. In: Page AL, Miller RH, Keeney DR (eds) Methods of soil analysis, Part 2. Chemical and Microbiological Properties. Agronomy Monograph No. 9. ASA-SSSA, Madison, Wisconsin, USA, pp 595–624
Brewer LJ, Sullivan DM (2003) Maturity and stability evaluation of composted yard trimmings. Compost Sci Util 11(2):96–112
Article Google Scholar
Bundela PS, Gautam SP, Pandey AK, Awasthi MG, Sarsaiya S (2010) Municipal solid waste management in Indian cities—a review. Int J Environ Sci 1(4):591–606
Carr L, Grover R, Smith B, Richard T, Halbach T (1995) Commercial and on-farm production and marketing of animal waste compost products. In: Steele K (ed) Animal waste and the land–water interface. Lewis Publishers, Boca Raton, pp 485–492
Community Development Research (2011) Ethiopia solid waste and landfill (country profile and action plan). Global Methane Initiative http://www.globalmethane.org/ . Accessed on August 2012
Cooperband LR, Stone AG, Fryda MR, Ravet JL (2003) Relating compost measures of stability and maturity to plant growth. Compost Sci Util 11(2):113–124
Delgado M (2010) Phytotoxicity of uncomposted and composted poultry manure, African. J Plant Sci 4:154–162
Dias BO, Silva CA, Higashikawa FS, Roig A, Sánchez-Monedero MA (2010) Use of biochar as bulking agent for the composting of poultry manure: effect on organic matter degradation and humification. Bioresour Technol 101:1239–1246
Domínguez J, Edwards CA (2010) Relationships between composting and vermicomposting: relative values of the products. In: Edwards CA, Arancon NQ, Sherman RL (eds) Vermiculture technology: earthworms, organic waste and environmental management. CRC Press, Boca Raton, pp 1–14
Dominguez J, Edwards CA, Subler S (1997) Comparison of vermicomposting and composting. Bio-Cycle 38(4):57–59
CAS Google Scholar
Dumitrescu L, Manciulea I, Sauciuc A, Zaha C (2009) Obtaining fertilizer compost by composting vegetable waste, sewage sludge and sawdust. In: Bulletin of the Transilvania, vol 2, no 51. University of Braşov, pp 117–122
Eastman BR, Kane PN, Edwards CA, Trytek L, Gunadi B, Stermer AL, Mobley JR (2001) The effectiveness of vermiculture in human pathogen reduction for USEPA biosolids stabilization. Compost Sci Util 9(1):38–49
Edward CA, Bohlen PJ (1996) Biology and ecology of earthworms. Chapman and Hall, London
Edwards CA, Subler S (2011) Human pathogen reduction during vermicomposting. In: Edwards CA, Arancon NQ, Sherman R (eds) Vermiculture technology Florida. CRC Press Taylor and Francis Group, Florida, pp 249–261
Epstein E (1997) The science of composting. Technomic Publishing Company Inc, Lancaster
Finstein MS, Miller FC, Strom PF (1986) Monitoring and evaluating composting process performance. J Water Pollut Control Fed 58(4):272–278
Forster JC, Zech W, Wiirdinger E (1993) Comparison of chemical and microbiological methods for the characterization of the maturity of composts from contrasting sources. Biol Fertil Soils 16:93–99
Frederickson J, Butt KR, Morris RM, Daniels C (1997) Combining vermiculture with traditional green waste composting systems. Soil Biol Biochem 29(3/4):725–730
Frederickson J, Howell G, Hobson AM (2007) Effect of pre-composting and vermicomposting on compost characteristics. Eur J Soil Biol 43:S320–S326
Gallizzi K (2003) Co-composting reduces helminth eggs in faecal sludge: a field study in Kumasi, Ghana. SANDEC, Dübendorf, p 45
Gantzer C, Gaspard P, Galvez L, Huyard A, Dumouthier N, Schwartzbrod J (2001) Monitoring of bacteria and parasitological contamination during various treatment of sludge. Water Resour 35(16):3763–3770
Gao M, Liang F, Yub A, Li B, Yang L (2010) Evaluation of stability and maturity during forced-aeration composting of chicken manure and sawdust at different C/N ratios. Chemosphere 78:614–619
Garcia C, Hernandez T, Costa F (1993) Evaluation of the organic matter composition of raw and composted municipal wastes. Soil Sci Plant Nutr 39:99–108
Gómez-Brandón M, Lazcano C, Domínguez J (2008) The evaluation of stability and maturity during the composting of cattle manure. Chemosphere 70:436–444
Guo R, Li G, Jiang T, Schuchardt F, Chen T, Zhao Y, Shen Y (2012) Effect of aeration rate, C/N ratio and moisture content on the stability and maturity of compost. Bioresour Technol 112:171–178
Haq T, Ali TA, Begum R (2014) Seed germination bioassay using maize seeds for phytoxicity evaluation of different composted materials. Pak J Bot 46(2):539–542
Hill GB, Lalander C, Baldwin SA (2013) The effectiveness and safety of vermi-versus conventional composting of human feces with Ascaris suum ova as model helminthic parasites. J Sustain Dev 6(4):1–10
Hock LS, Baharuddin AS, Ahmed MN, Md. Shah UK, Abdul Rahaman NA, Abd-Aziz S, Hassan MA, Shirai Y (2009) Physicochemical changes in windrow co-composting process oil palm mesocarpfiber and palm oil effluent anaerobic sludge. Aust J Basic Appl Sci 3(3):2809–2819
http://www.cfe.cornell.edu/compost/invertebrates.html (1 of 4) [1/16/2001 8:49:10 AM]. Cornell composting Science and Engineering
Huang GF, Fang M, Wu QT, Zhou LX, Liao XD, Wong JWC (2001) Co-composting of pig manure with leaves. Environ Technol 22:1203–1212
Iqbal MK, Khan RA, Nadeem A, Hussnain A (2012) Comparative study of different techniques of composting and their stability evaluation in municipal solid waste. J Chem Soc Pak 34(2):273–282
Jusoh ML, Manaf LA, Abdul Latif P (2013) Composting of rice straw with effective microorganisms (EM) and its influence on compost quality. Iran J Environ Health Sci Eng 10:17
Khwairakpam M, Kalamdhad AS (2011) Vermicomposting of vegetable wastes amended with cattle manure. Res J Chem Sci 1(8):49–56
Koné D, Cofie O, Zurbru C, Gallizzi K, Moser D, Drescher S, Strauss M (2007) Helminth eggs inactivation efficiency by faecal sludge dewatering and co-composting in tropical climates. Water Res 14(9):4397–4402
Koné D, Cofie O, Nelson K (2010) Low-cost options for pathogen reduction and nutrient recovery from faecal sludge. In: Drechsel P, Scott CA, Raschid-Sally L, Redwood M, Bahri A (eds) Wastewater irrigation and health: assessing and mitigating risk in low-income countries. International Water Management Institute (IWMI), Earthscan, International Development Research Centre (IDRC), Colombo, pp 171-188.
Kumar R, Shweta (2011) Removal of pathogens during vermi-stabilization. J Environ Sci Technol 4(6):621–629
Kumar PR, Jayaram A, Somashekar RK (2009) Assessment of the performance of different compost models to manage urban household organic solid wastes. Clean Technol Environ Policy 11:473–484
Lazcano C, Gómez-Brandón M, Domínguez J (2008) Comparison of the effectiveness of composting and vermicomposting for the biological stabilization of cattle manure. Chemosphere 72:1013–1019
Levanon D, Pluda D (2002) Chemical, physical and biological criteria for maturity in composts for organic farming. Compost Sci Util 10(4):339–346
Lung AJ, Lin CM, Kim JM, Marshall MR, Nordstedt R, Thompson NP, Wei CI (2001) Destruction of Escherichia coli O157:H7 and Salmonella enteritidis in cow manure composting. J Food Prot 64:1309–1314
Maso MA, Blasi AB (2008) Evaluation of composting as a strategy for managing organic wastes from a municipal market in Nicaragua. Bioresou Technol 99:5120–5124
Monroy F, Aira M, Domínguez J (2008) Changes in density of nematodes, protozoa and total coliforms after transit through the gut of four epigeic earthworms (Oligochaeta). Appl Soil Ecol 39:127–132
Mupondi LT, Mnkeni PNS, Brutsch MO (2006) The effects of goat manure, sewage sludge and effective microorganisms on the composting of pine bark. Compost Sci Util 14:201–210
Mupondi LT, Mnkeni PN, Muchaonyerwa P (2010) Effectiveness of combined thermophilic composting and vermicomposting on biodegradation and sanitization of mixtures of dairy manure and waste paper. Afr J Biotechnol 9(30):4754–4763
Ndegwa PM, Thompson SA (2001) Integrating composting and vermicomposting in the treatment and bioconversion of solids. Bioresour Technol 76:107–112
Niwagaba C, Nalubega M, Vinnerås B, Sundberg C, Jonsson H (2009) Benchscale composting of source-separated human faeces for sanitation. Waste Manag 29:585–589
Okalebo JR, Guthua KW, Woomer PJ (2002) Laboratory methods of soil and plant analysis—a working manual. TSBF-CIAT and SACRED Africa, Nairobi
Padmavathiamma PK, Li LY, Kumari UR (2008) An experimental study of vermin biowaste composting for agricultural soil improvement. Bioresour Technol 99:1672–1681
Pisa C, Wuta M (2013) Evaluation of composting performance of mixtures of chicken blood and maize stover in Harare, Zimbabwe. Int J Recycl Org Waste Agric 2(5):1–11
Plym-Forshell L (1995) Survival of Salmonellas and Ascarissuum eggs in a thermophilic biogas plant. Acta Vet Scandinavica 36:79–85
Rao KJ (2007) Composting of municipal and agricultural wastes. In: Proceedings of the international conference on sustainable solid waste management, Chennai, India, 5–7 September 2007
Rodriguez-Canche LG, Cardoso-Vigueros L, Maldonado-Montiel T, Martinez Sanmiguel M (2010) Pathogen reduction in septic tank sludge through vemicomposting using Eisenia fetida . Bioresour Technol 101:3548–3553
Schönning C, Stenström TA (2004) Guidelines for the safe use of urine and faeces in ecological sanitation. Report 2004-1. Ecosanres, SEI. Sweden. www.ecosanres.org
Schwartzbrod J (2003) Quantification and viability determination for helminth eggs in sludge (modified EPA method 1999). University of Nancy, Nancy
Seal A, Bera R, Chatterjee AK, Dolui AK (2012) Evaluation of a new composting method in terms of its biodegradation pathway and assessment of compost quality, maturity and stability. Arch Agron Soil Sci 58(9):995–1012
Selim SM, Zayed MS, Atta MH (2012) Evaluation of phytotoxicity of compost during composting process. Nat Sci 10(2):69–77
Smith DC, Hughes JC (2002) Changes in chemical properties and temperature during the degradation of organic wastes subjected to simple composting protocols suitable for small-scale farming, and quality of the mature compost. S Afr J Plant Soil 19:53–60
Soares MR, Matsinhe C, Belo S, Quina MJ, Quinta-Ferreira R (2013) Phytotoxicity evolution of biowastes undergoing aerobic decomposition. J Waste Manag. doi: 10.1155/2013/479126
Soumaré M, Demeyer A, Tack FMG, Verloo MG (2002) Chemical characteristics of Malian and Belgian solid waste composts. Bioresour Technol 81:97–101
Strauch D (1991) Survival of pathogenic micro-organisms and parasites in excreta, manure and sewage sludge. Revue Sci Tech (Int Off Epizoot) 10:813–846
Sundberg C, Smars S, Jonsson H (2004) Low pH as an inhibiting factor in the transition of mesophilic to thermophilic phase in composting. Bioresour Technol 95:145–150
Tiquia SM, Tam NFY (1998) Elimination of phytotoxicity during co-composting of spent pig-manure sawdust litter and pig sludge. Bioresour Technol 65:43–49
Tiquia SM, Richard TL, Honeyman MS (2002) Carbon, nutrient, and mass loss during composting. Nutr Cycl Agroecosyst 62:15–24
Tognetti C, Loas F, Mazzarino MJ, Hernandez MT (2005) Composting vs. vermicomposting: a comparison of end product quality. Compost Sci Util 13(1):6–13
Tognetti C, Mazzarino MJ, Laos F (2007) Improving the quality of municipal organic waste compost. Bioresour Technol 98:1067–1076
USEPA (United States Environmental Protection Authority) Pathogen Equivalency Committee (PEC) (1999) Control of pathogens and vector attraction in sewage sludge. In: USEPA Environmental Regulations and Technology, Office of Research and Development EPA/625/R-92/013, Washington, DC, p 177
Vinnerås B (2007) Comparison of composting, storage and urea treatment for sanitising of faecal matter and manure. Bioresour Technol 98:3317–3321
Vuorinen AH, Saharinen MH (1997) Evolution of microbiological and chemical parameters during manure and straw co-composting in a drum composting system. Agric Ecosyst and Environ 66:19–29
Walkley A, Black IA (1934) An examination of the Degtjareff method for determining soil organic matter and proposed modification of the titration method. Soil Sci Soc Am J 37:29–34
WHO (2006) Guidelines for the safe use of wastewater, excreta and greywater, vol 4. Excreta and grey water use in agriculture. ISBN: 92 4 154685 9
Witter E, Lopez-Real J (1988) Nitrogen losses during the composting of sewage sludge, and the effectiveness of clay soil, Zeolite and compost in adsorbing volatilised ammonia. Biol Wastes 23:279–294
Wu L, Ma LQ, Martinez GA (2000) Comparison of methods for evaluating stability and maturity of bio-solids compost. J Environ Qual 29(2):424–429
Xanthoulis D, Strauss M (1991) Reuse of wastewater in agriculture at Ouarzazate, Morocco (Project UNDP/FAO/WHO MOR 86/018). Unpublished mission reports
Yadav KD, Tare V, Ahammed MM (2010) Vermicomposting of source separated human faeces for nutrient recycling. Waste Manag 30:50–56
Yadav KD, Tare V, Ahammed MM (2012) Integrated composting–vermicomposting process for stabilization of human faecal slurry. Ecol Eng 47:24–29
Zmora-Nahum S, Markovitch O, Tarchitzky J, Chen Y (2005) Dissolved organic carbon (DOC) as a parameter of compost maturity. Soil Biol Biochem 37:2109–2116
Zucconi F, de Bertoldi M (1987) Compost specification for the production and characterization of compost from municipal solid waste. In: de Bertoldi M, Ferranti MP, Hermite PL, Zucconi F (eds) Compost: production, quality and use. Elsevier Applied Science Publishers, Barking, pp 30–50
Zucconi F, Forte M, Monaco A, De Bertoldi M (1981) Biological evaluation of compost maturity. Biocycle 22(4):27–29
Download references
Authors’ contributions
TM conceived and carried out the study; performed the analyses and drafted the manuscript. HG participated in the design of the study; KK, KW, BS and HY participated in the design of the study supervised the analysis process and helped draft the manuscript. All authors read and approved the final manuscript.
Acknowledgements
This study was financially supported by the Ministry of Education of the Federal Democratic Republic of Ethiopia. Authors wish to thank the Sanitation and Beautification Agency (SBA) and Water Supply and Sewerage Authority (WSSA) of Dire Dawa City Administration for their cooperation in collecting and providing the compostable material.
Competing interests
The authors declare that they have no competing interests
Author information
Authors and affiliations.
School of Natural Resources Management and Environmental Sciences, Haramaya University, P.O.Box 138, Dire Dawa, Ethiopia
Tesfu Mengistu, Heluf Gebrekidan, Kibebew Kibret, Beneberu Shimelis & Hiranmai Yadav
School of Plant Sciences, Haramaya University, P.O.Box 138, Dire Dawa, Ethiopia
Kebede Woldetsadik
You can also search for this author in PubMed Google Scholar
Corresponding author
Correspondence to Tesfu Mengistu .
Additional file
Additional file 1. table s1 mean daily temperature values of different composting methods, rights and permissions.
Open Access This article is distributed under the terms of the Creative Commons Attribution 4.0 International License ( http://creativecommons.org/licenses/by/4.0/ ), which permits unrestricted use, distribution, and reproduction in any medium, provided you give appropriate credit to the original author(s) and the source, provide a link to the Creative Commons license, and indicate if changes were made.
Reprints and permissions
About this article
Cite this article.
Mengistu, T., Gebrekidan, H., Kibret, K. et al. Comparative effectiveness of different composting methods on the stabilization, maturation and sanitization of municipal organic solid wastes and dried faecal sludge mixtures. Environ Syst Res 6 , 5 (2018). https://doi.org/10.1186/s40068-017-0079-4
Download citation
Received : 13 May 2016
Accepted : 01 January 2017
Published : 14 January 2017
DOI : https://doi.org/10.1186/s40068-017-0079-4
Share this article
Anyone you share the following link with will be able to read this content:
Sorry, a shareable link is not currently available for this article.
Provided by the Springer Nature SharedIt content-sharing initiative
- Faecal coliform
- Faecal sludge
- Helminth egg
- Municipal solid waste
- Sanitization
- Stabilization
- Vermicomposting
Composting: A Sustainable Route for Processing of Biodegradable Waste in India
- Open Access
- First Online: 24 November 2020
Cite this chapter
You have full access to this open access chapter
- Ashootosh Mandpe 4 ,
- Sweta Kumari 4 &
- Sunil Kumar 4
13k Accesses
7 Citations
Surging populations, coupled with the ever-increasing demand for sustenance, have led to the generation of behemoth proportions of wastes throughout the globe. The processing of such a considerable amount of waste has raised concerns for environmental planners, policymakers, and researchers in regard to maintaining sustainability. Biodegradable waste is a part of the total waste stream. Consideration should be given to the importance of making better use of biodegradable waste. The technology that is adopted for the management of biodegradable waste should be ecologically sustainable and cost-effective, as well as beneficial to social well-being. The most efficient way of managing biodegradable waste must include different methods for the optimal utilisation of such waste, ranging from the small scale (single household) to the very large scale (entire city). Amid all the other waste processing technologies, composting stands out as a most potent option because of its ability to maintain and restore soil fertility, along with the transformation of waste into a resource. Composting is one of the few technologies which has a benefit–cost ratio higher than 1 at all scales of operation. This chapter analyses the most significant aspects of the composting process, including the recent developments and dynamics involved in it. The chapter discusses various aspects of composting via analysis of the integrated waste management system and composting-related projects implemented at the community level in the Indian context. Finally, the chapter presents policies and the efforts put in place by the Government of India with the aim of encouraging composting practice and related activities.
You have full access to this open access chapter, Download chapter PDF
Similar content being viewed by others
Composting: An Alternative with Marked Potential for Organic Waste Management
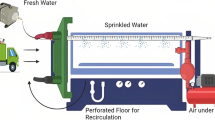
From waste to wealth: exploring modern composting innovations and compost valorization
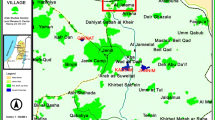
Organic waste composting and sustainability in low-income communities in Palestine: lessons from a pilot project in the village of Al Jalameh, Jenin
- Biodegradable waste
- Biological decomposition
- Community-based implementation
- Composting techniques
- Municipal solid waste (MSW) management
- Sustainable utilisation
1 Introduction
The swelling population, along with ever-increasing demands, has led to the generation of behemoth volumes of waste. The waste generation rate is proportional to the human population throughout the globe. Management of these enormous volumes of generated waste tends to put pressure on urban local bodies governing the cities or the urban parts of the Indian country. In the twenty-first century, solid waste management has gained significant consideration from various environmental planners, policymakers, non-governmental organisations and waste managers because of the negative environmental impacts resulting from unscientific management and adoption of unsafe disposal practices in respect of waste. The situation is particularly distressing in developing countries: municipal solid waste (MSW) can be spotted almost ubiquitously in the urban as well as semi-urban parts of most developing countries. Eco-friendly MSW management has become a challenging task in India due to the increasing population, unparalleled and unalterable urbanisation, as well as industrial development (Ramachandra et al. 2018 ). To give an idea of the size of the problem, Table 3.1 presents today’s MSW generated per capita in India and other developing countries, as well as the forecast figures (Hoornweg and Bhada-Tata 2012 ).
MSW poses a considerable risk to the surrounding environment, including water, soil, and human health. According to the Central Pollution Control Board (CPCB) report, the MSW in India has significantly higher fraction of organics (CPCB 2013a ). Enormous volumes of biodegradable solids get generated as earthly and aquatic weeds, leaf litter, and agricultural waste. The weeds, if left untreated, may infest the land and water resources and may lead to their depletion. In developing nations, the practice of burning agricultural waste and leaf litter in the open air is still prevalent, which not only abolishes the significant deal regarding carbon and other nutrients but also causes air pollution, resulting in global warming.
According to Tomić and Schneider ( 2017 ), different approaches have been adopted as alternatives to traditional methods of solid waste management. These include the reuse of MSW and biodegradables waste, which is done via thermal processing and bioprocessing techniques. Thermal processing techniques comprise incineration, pyrolysis, and gasification; however, these do not seem feasible due to the low calorific values (800–1000 kcal/kg for Indian MSW) of MSW generated in developing countries, high energy intensiveness, as well as the fact that they result in other environmental hazards. Bioprocessing techniques comprise anaerobic digestion and composting. Among all other waste processing technologies, composting seems to be a powerful option because of its ability to maintain and restore soil fertility, along with the transformation of waste into a resource. Composting is among the best known processes for the biological stabilisation of biodegradable waste and is one of the few technologies which has a benefit–cost ratio greater than 1 for all scales of operation.
Composting can be explained as the process of transforming the organic substrate into nutrient-rich manure through the medium of microbial communities. The prerequisite of the composting process is the availability of substrate in an organic origin (Gajalakshmi and Abbasi 2008 a). More precisely, composting signifies the process of the biodegradation of the mixture of organic substrate conducted by the populations of various microbial species in aerobic environments in the solid state.
The process of composting generally takes place in four phases, and they may occur concomitantly, rather than sequentially (Belyaeva and Haynes 2009 ). These four phases include:
The mesophilic phase
The thermophilic phase
The cooling phase
The curing phase
During the initial phase (the mesophilic phase), which is also termed the decomposition phase, the bacterial community present in the mixture of organic substrate combines oxygen with carbon to produce energy and carbon dioxide. A portion of the energy is utilised by the microorganisms for growth and reproduction processes, and the rest is released as heat. In this stage, the oxidation of easily degradable organic matter takes place, along with the proliferation of mesophilic bacteria, resulting in a rise in temperature of the substrate to be composted. These mesophilic bacteria may include E. coli and other bacterial strains which are inhibited by the temperature as the process is taken over by the thermophilic bacteria in the transition range.
The next phase, i.e. the thermophilic phase, which is also termed the stabilisation phase, involves the mineralisation of slowly degradable molecules, along with complex process like humification of lignocellulosic compounds. In this stage, a rise in temperature can be observed, which lasts only for a few days. After the completion of a thermophilic phase, the available manure seems to be digested, but the bristlier materials will remain intact. This phase is the cooling phase, in which the mesophilic microbes, which were hurtled away during the thermophilic phase, take over the system and start digesting the more resilient organic constituents of the substrate. Fungi and other macroorganisms like earthworms also enter back into the system.
A long curing time acts as a safety net for the destruction of remaining pathogens. The immature compost can be detrimental to plants and may even result in the production of phytotoxins. These phytotoxins may deprive the soil of nitrogen and oxygen and can contain higher levels of organic acids (Vandergheynst 2009 ).
2 Composting of Different Wastes
Composting is a cost-effective and clean option for waste disposal. It also offers extra benefits such as reduction of greenhouse gas (GHG) emissions, space taken by the landfill sites, and groundwater and surface water contamination by waste. The reduction in GHG emission consequently helps in regard to the issue of global warming/climate change (Hubbe et al. 2010 ).
Composting is a fundamental feature of an integrated solid waste management strategy. For effective integrated solid waste management, the key approaches are reducing, reusing, recycling, and managing waste to ensure environmental and human health safety. Local situations and needs vary from region to region and so does the composting process. Composting is a part of organic recycling, which converts organic waste into a nutrient-rich soil conditioner and also results in a reduction of GHG emissions (Lou and Nair 2009 ). The composting of different waste substrates is discussed in brief in the following subsections.
2.1 Agricultural/Lignocellulosic Waste
To maintain sufficient and long-lasting humus, biodegradable wastes, such as sawdust, wood shavings, coir pith, pine needles, and dry fallen leaves, are mixed together (Gajalakshmi and Abbasi 2008 ). However, it is observed that lignin-rich plant materials do not disintegrate rapidly. To enhance the decomposition, the waste material is treated with lime. They are mixed in a ratio of 5 kg of lime per 1000 kg of waste to prepare a good compost from hard plants. Lime can be in the form of dry powder or semi-solid substance when mixed with water. Liming weakens the lignin structure and enhances the humification process in plant residues, thus improving the humus quality (Hubbe et al. 2010 ). As an alternative to lime, phosphate rock in a dry powder state can be mixed in a ratio of 20 kg per 1000 kg of organic waste; it contains phosphates and micronutrients, which make a compost that is rich in plant nutrients.
2.2 Sewage Sludge
Sludge from sewage can be mixed with carbon by-products from agricultural sources, such as straw, sawdust, or wood chips, to produce compost. Microorganisms and parasites, which can cause diseases, are killed by the heat generated from bacteria digesting both sewage sludge and plant material in the presence of oxygen. Proper air circulation is obtained by maintaining aerobic conditions under 10–15% oxygen and bulking agents like shredded tyres. Stiff materials separated from softer leaves and lawn clippings maintain better ventilation (Fang et al. 1999 ).
An insulating blanket of previously composted sludge is placed over aerated composting piles for uniform distribution of pathogen-killing temperatures. The composting mixture should have an initial moisture level of about 50%, but if the temperature is inadequate for pathogen reduction, then the compost moisture content rises above 60%. Hence, composting mixtures piled on concrete pads with built-in air ducts might improve air circulation (Fang et al. 1999 ). A blower drawing vacuum may be used to minimise odours. When the moisture reaches 70%, exhausting through filtering pile can be replaced. The accumulated liquid in the underdrain ducting may be returned to the sewage treatment plant. For better moisture control, roofing of composting pads is required.
After a small interval that is sufficient for pathogen reduction, undigested bulking agents are recovered for reuse. The optimum initial carbon-to-nitrogen ratio of a composting mixture is between 26:1 and 30:1, but the amount required to dilute concentrations of toxic chemicals in the sludge to acceptable levels for the intended compost use determines the composting ratio of agricultural by-products. Agricultural by-products have less toxicity; however, suburban grass clippings containing residual herbicide levels are detrimental to some agrarian purposes, and freshly composted wood by-products may contain phytotoxins that inhibit the germination of seedlings until detoxified by soil fungi (Jouraiphy et al. 2005 ).
Composting yard trimmings, agricultural wastes, and sewage sludge have increased the interest in composting of organic fractions from MSW. MSW contains materials that vary in size, moisture, and nutrient content, and the organic fractions can be mixed with varying degrees of non-compostable wastes and possibly hazardous constituents. Producing a market-savvy compost product requires a range of physical processing technologies, in addition to the biological process management (Varma and Kalamdhad 2013 ).
Modern MSW composting system consists of four tasks: collection, contaminant separation, sizing and mixing, and biological decomposition. The collection task is representative of the characteristics of the incoming waste, which largely determines the processing requirements of the remaining tasks. The separation task generates recyclable and rejects streams. Biological activity can be enhanced by increasing the surface area of the waste in size reduction, whereas mixing ensures the adequacy of nutrients, moisture, and oxygen in the material.
Evaluation of system design: Several criteria are important, like cost (capital, operations, and maintenance), market specifications for compost and recyclable by-products, and system flexibility to respond to a changing MSW feedstock. The economic analysis of a composting facility should evaluate options like landfilling or incineration, along with different ways of achieving the same goal. It should also include analysis of cost between source separation, centralised separation, contaminant removal, and market assessment for recyclable by-product streams and the compost product (Wei et al. 2017 ). A composting facility should consider the quality control and product, as it might be a problem if a particular technology produces a less recyclable product as compared to markets. A flexible facility will be able to adapt to changes in the regulatory environment, in market specifications, and in the waste stream. Hence, the MSW facility should be flexible, in order to operate in the long term.
2.4 Biomedical Waste
Biomedical waste needs to be pre-treated with 5% sodium hypochlorite (NaOCl) at the disposal site for safety measures (Dinesh et al. 2010 ). It should be subjected to an initial decomposition process by blending it with cow dung slurry and can be further treated using the vermicomposting technique. Various epigeal species of worms can be utilised for this purpose. Practising this methodology for treating biomedical wastes can make these worms more efficient in carrying out the decomposition process. Vermicomposting with proper handling of biomedical waste can be an energy-efficient, eco-friendly approach for reducing and recycling this hazardous waste (Dinesh et al. 2010 ).
3 Composting Techniques Used in India
The organic fraction comprises the significant portion of waste generated in developing countries like India, and due to improper waste management practices, only a minor portion of the total waste volume is processed. The composting technique has been divided into two categories: the first category comprises conventional composting techniques, while the other category includes novel composting techniques. Each of the composting techniques is explained in brief in the following section. Figure 3.1 shows different composting techniques adopted for processing various types of biodegradable waste.

Different composting techniques practised in India
3.1 Conventional Composting Techniques
Windrow composting.
In a windrow composting system, piles of waste are kept for the decomposition and aeration activity and are turned simultaneously. The turning of waste piles leads to the lowering of the temperature of the compost and also allows the circulation of air in the system. Windrow systems are comparatively economical since there is no such requirement of any mechanical tools for providing aeration. The height of the windrow depends on the type of waste substrate and the equipment or tool used for flipping purposes. The intermittent flipping of the waste heaps is necessary for maintaining the optimal temperature (Kumar 2011 ).
Aerated Static Pile Composting
In an aerated static pile composting system, the waste substrate is decomposed without any physical activity over 30–35 days. Perforated pipes are used for providing aeration to the waste piles. These waste piles can be kept open, covered, semi-covered, or in windrow form. The rate of aeration is one of the most critical factors in this type of composting system. The asperity of aeration produces the vertical temperature difference within the entire structure of the waste pile (Hubbe et al. 2010 ).
Vermicomposting
The process of vermicomposting involves the utilisation of earthworms of different species for the disintegration of semi-decomposed biodegradable waste. It has been found that the earthworms can consume up to five times their body mass every day. According to CPCB (CPCB 2013b ) report, the total number of composting and vermicomposting plants located in several states of India are shown in Fig. 3.2 . Earthworm species commonly used in the vermicomposting systems are Perionyx excavatus , Eudrilus eugeniae , and Eisenia foetida . These epigeal species are extremely prolific feeders which can devour diverse streams of organic waste.
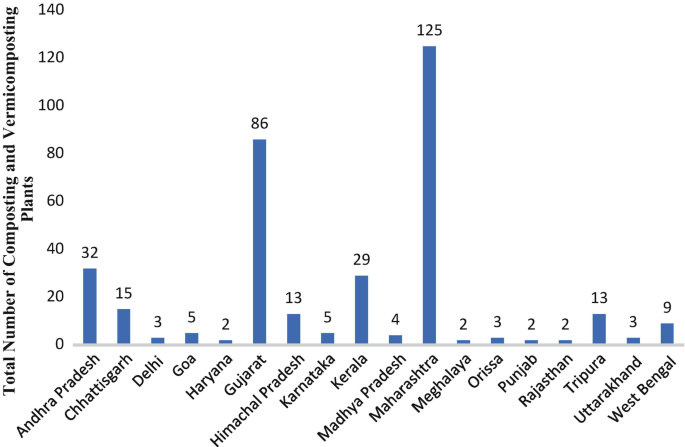
Total number of composting and vermicomposting plants across India. (Source: CPCB 2013b )
Aerobic Composting
The aerobic composting system is carried out in the presence of air at a specific temperature and pH range where the microbial consortia decompose the biodegradable waste into a nutrient-rich bio-fertiliser (Monson and Murugappan 2010 ). During the aerobic composting process, the optimal rate of aeration can solve the problem of odours.
In-vessel Composting
The in-vessel composting system involves the decomposition of the organic fraction of the waste in a closed container or vessel in a controlled environment (Manyapu et al. 2017 ). Various in-vessel composting systems comprise rotating drums and agitated bags. Instinctive aeration and mechanical agitation can enhance the decomposition process and boost the rapid composting process. Motorised agitation may lead to contravention of particles, providing a healthier contact of the microorganisms with the carbon, while the higher temperature in the vessels can finish the pathogenic activities in the system, and an unceasing aeration results in the removal of odours from the system (Monson and Murugappan 2010 ).
3.2 Novel Composting Techniques
To overcome the problems associated with conventional composting practices and to improve the efficiency of the composting system, different researchers and environmentalists throughout the globe have adopted various approaches for effectual composting of biodegradable waste; these are termed novel composting techniques in this chapter. One of the approaches, called the co-composting technique, involves the utilisation of different waste substrates; another approach involves utilisation of arthropods like millipedes, black soldier flies, and similar organisms for composting purposes. Both of these techniques are explained in brief in this section.
Co-composting of Different Waste Substrates
This is a technique in which two different waste substrates are mixed together and applied to augment the composting activity. Co-composting of the waste substrate using specific type of bulking agent can offer sufficient particle structure and the denseness of the particles may tend to provide airspace needed for improving the aerobic microbial activity, leading to an increased rate of biodegradation. Various researchers have utilised food waste as a substrate for co-composting with other waste substrates because of their unique characteristics. Practising the co-composting of food waste can prove to be the best solution for processing food waste. Food waste can be blended with other waste substrates like chopped hay, rice husks, wooden chips, wheat straw, rice bran, sawdust, and other similar biodegradable waste to maintain the favourable carbon/nitrogen (C/N) ratio, void spaces, moisture, and nitrogen content. The problem regarding the lower pH of the food waste can be solved by adding sodium acetate in the mixture of the waste, to balance the pH level of the system. Similarly, different feedstocks, like pig manure, poultry litter, etc., can be applied for co-composting activities with other supplementary waste substrates (Kumar et al. 2018 ).

Composting Using Arthropods
Among the different techniques practised for the management of biodegradable waste, fertilising the soil is the most favoured one. In recent years, novel techniques have been developed for efficient composting of biodegradable waste, and various arthropods have been used in the composting process. Many species of insects are efficiently utilised for reprocessing and transforming waste of vegetal origin. The blends of different types of waste formed at diverse stages of decomposition entice an essential species of arthropods whose life cycle is over in the compost, while any further addition to it enhances the decomposition process. These arthropodal species boost the development of the consentient species (Kumar et al. 2018 ). Arthropods like millipedes and black soldier fly larvae have been successfully used by Karthigeyan and Alagesan ( 2011 ) and Diener et al. ( 2015 ) for the composting of different types of organic waste. The millipedes transform the waste substrate into faecal pellets, which affect the required physicochemical characteristics by decreasing the C/N ratios (Karthigeyan and Alagesan 2011 ).
4 Composting as a Technique for Solid Waste Management in the Indian Scenario
Like other developing countries, India is also confronting solid waste management as one of the major issues it faces. Due to the expeditious growth in population, urbanisation, and industrialisation, India is switching towards an industrial and service-based economy and away from an agricultural-based economy. India has 29 states and 7 union territories and has climatic, geographical, ecological, cultural, traditional, and religious heterogeneity. The present urban population of India accounts for 31.2% (377 million) of its total population (Census 2011 ). As per CPCB (CPCB 2013b ), India generated about 127,486 tonnes per day (TPD) of waste in 2011, out of which 89,334 TPD (70%) was collected; only 15,881 (12.45%) of the total waste was processed (CPCB 2013b ). Being a developing country, the organic fraction of MSW in India is usually high. The urban MSW composition in Indian cities contains approximately 40% organic, 40% inert, and 19% of recyclable fractions (Sharholy et al. 2008 ). India has 279 composting plants, 138 vermicomposting facilities, 172 bio-methanation processors, 29 pelletisation plants, and 34 landfill sites across the country (Planning Commission 2014 ). From the numbers, it can be understood that composting technology is the most favoured and recommended among all the other technologies for efficient waste management. The problem of waste management is still prevalent in the country and is one of the major concerns for urban local bodies, which govern the urban parts of the country. In spite of the facilities above, it is believed that 90% of waste in India is dumped in an unscientific manner (Gajalakshmi and Abbasi 2008 ). The average per capita waste generated ranges from approximately 0.17 to 0.62 kg depending on location, economic activity, and culture (Kumar et al. 2009 ). The rapid composting technique is highly encouraged in big Indian cities. Indore composting centre is one of the foremost maintained composting plants in the country. Considering different zones in India, the following subsections discuss the current scenario of composting in several cities, along with the overall scenario of composting on the ground level.
4.1 Composting in the City of Kolkata
Kolkata city is the capital of the state of West Bengal. According to the South Asian Forum for the Environment, Kolkata generates 4000 tonnes of waste per day, out of which 37% is compostable (Dutta 2018 ). The major issue being faced by the Kolkata Municipal Corporation is the unavailability of land. The only landfill site in the region is “Dhapa”, and it is already oversaturated from use over the last 30 years. The Dhapa dumpsite is located in the East Kolkata Wetlands, which is a Ramsar Convention Wetland. Dhapa dumpsite alone contributes to 7500 cubic metres of GHGs per hour (Times News Network 2013 ). According to a news daily, Kolkata city needs at least two solid waste treatment plants for the efficient management of waste (Dutta 2018 ). Keeping in view the massive problem of waste management, many housing complexes and societies have started installing compost machines within their premises for the treatment of MSW generated from household activities. The Belani Group of Companies, which is a housing development firm, has installed two composting facilities near the Hiland Riverside project in Batanagar. It has been announced that the Kolkata Municipal Corporation will be installing a similar composting facility in Tollygunge, Madhyamgram, and Rajarhat. These areas are among the most densely populated regions in Kolkata city and are also being developed at a rapid pace. Over 1100 apartments in South City (Residential Complex) in Kolkata have also joined this movement and have decided to sensitise the MSW for soon implementing the same mechanism in their housing complexes (Desai 2018 ). A small town in West Bengal named Uttarpara has helped Kolkata in receiving a prestigious award under the Urban Solid Waste Management category in the C40 Mayors’ Summit held in Mexico on 1 December 2016, for its exclusive and effective solid waste management, which was mainly focussed on the segregation of waste at source. It was found that all the waste in Uttarpara is separated at source into biodegradable and non-biodegradable categories (Rao 2016 ). It has been reported that, earlier, a dumpsite, with debris ranging up to 50 ft. in length, was present in the town. However, a composting facility has been installed there and is operating successfully (Bhattacharya 2016 ). Nowadays, the composting facility at Uttarpara receives about 12–14 tonnes of raw biodegradable waste and produces 3 to 4 tonnes of manure per day (Rao 2016 ). The attitude of negligence towards the dumping site in Kolkata resulted in deplorable hygiene conditions and health impacts. Kolkata is a metropolitan city which is gradually moving towards a clean society. Small steps may gradually change the current scenario, but at the same time, the pace needs to be boosted to overcome the surging waste management problems.
Similarly, to promote sustainable waste management and utilise the nutrient-rich manure from composting processes, two female entrepreneurs from Kolkata, namely, Avantika Jalan and Rashmi Sarkar, have created a social enterprise called Mana Organics to recover soil fertility by producing chemical-free organic compost. Currently, they are operating four small composting projects: one in Tinsukia city, situated in the state of Assam, and the other three in villages in Madhya Pradesh. The compost produced from these composting facilities is marketed and sold in New Delhi and Kolkata. The firm started with a capital of 33.75 Lacs Rupees in 2011 (Basu 2014 ). Both entrepreneurs were inspired by their visit to Khargone district in the state of Madhya Pradesh. During their visit, they observed that a few regions in that district were devastated due to the failure of the cotton crop, and so they decided to help in solving the problem of the farmers (Basu 2014 ). The composting technique was adopted to manage the organic waste generated by agricultural and household activities to produce chemical-free organic manure, and they also spread awareness about the same among the farmers. Today, Mana Organics works with Adivasi (tribal) farmers in three villages of Madhya Pradesh: Sultanpur, Lachera, and Koriyakal (Basu 2014 ).
4.2 The Scenario of Composting in Delhi
Delhi is a union territory and the capital of India, and its present population is 11 million (Census 2011 ). According to a renowned environmental magazine, Down to Earth , Delhi generates 9500 TPD of waste, out of which 8000 TPD is collected and transported to three landfill sites, namely, Bhalswa, Ghazipur, and Okhla (Mudgal 2015 ). In some localities of the city, like Dwarka, Defence Colony, Sarita Vihar, Preet Vihar, and Dilshad Garden, composting facilities are already installed in their respective community parks, and segregation at source at the domestic level is being practised. The chairman of Delhi Municipal Corporation, Mr. Naresh Kumar, claimed that five localities in the state would become self-sustainable and would be transformed into a zero waste discharge site by the end of 2018 (Mudgal 2015 ). These five North Delhi Municipal localities are Pandara Park, Jor Bagh, Kaka Nagar, Bapa Nagar, and Golf Links. This idea was proposed in collaboration with a local resident welfare association. This plan emphasised segregation at source and separation into the wet waste, dry waste, and e-waste categories (Halder 2017 ). Delhi has three centralised composting plants at Narela, Bhalswa, and Okhla, which process around 500 TPD of biodegradable waste. Temples in Delhi are also coming forward to contribute to green practices. In Jhandewalan Temple, 5000–10,000 devotees visit every day, which results in the generation of 200 kgs of floral waste per day. On Tuesdays and Sundays, the waste generation rises to 500 kg, and during Navratri Puja (Goddess Festival), it goes up to about 1 TPD (Desai 2018 ).
In 2013 Delhi University invested 4 Lacs Rupees in the renowned college “Miranda House” to encourage composting within its premises to manage biodegradable waste most efficiently. The institute uses the raw material, comprising a mixture of horticulture and kitchen waste in a 1:3 ratio, for composting. The period required for composting is about 12–15 days (Mudgal 2015 ). The institute collaborated with Green Bandhu Environmental Solutions & Services, and now they produce 60 kgs of organic compost every day. It is encouraging the use of compost for recreational purposes in the region. The institute also earns around 4000–5000 Rupees by selling the supplementary compost produced from the composting plant and ultimately saves 12,000 Rupees which would have been incurred in the transport of waste (Mudgal 2015 ).
The General Pool Residential Complex in New Moti Bagh region is spread across 110 acres, with 1100 families. In 2013, this residential complex installed a composting facility provided by Green Planet Waste Management Pvt. Ltd. An area of 300 m 2 was needed for the composting facility, which receives around 700 kg of kitchen waste and 900 kg of horticultural waste per day (Mudgal 2015 ). The period required to get a matured compost is 15–20 days, thus producing organic compost. The company invested about 8 million Rupees in setting up this plant, and now the operators are struggling to recover the expense invested in installing the facility. A financial crisis or losses may result in its failure (Mudgal 2015 ).
The Defence Colony in the state of Delhi is practising composting for its kitchen waste using effective microorganisms (EM1) microbial solution-based pit composting. The composting period for this technology is relatively high, at 3–4 months. This setup requires a land area of just 30 m 2 , costing 70,000 Rupees only (Mudgal 2015 ). The cost for every household served by this plant is 45 Rupees only, which is quite affordable. This setup was established by the Residential Welfare Association (RWA), with the help of a non-profit organisation named Toxics Link. Two rag pickers are trained by the RWA to operate the compost facility, and their wages are paid for from the compost facility itself.
Recently, the North Delhi Municipal Corporation has declared that it will shortly set up four “bio-methanation” and “aerobic drum compost plants” under its jurisdictions. The bio-methanation plants are proposed to deal with green waste, having an intake capacity of 5 TPD, and the compost plants will have a capacity of 1 TPD load. These plants will deal with green waste generated from RWAs, parks, and vegetable markets. The total expenditure for 5 years for the procurement, operation, and maintenance of the plants would be a minimum of 17.95 Crores (Adak 2018 ).
4.3 Status of Composting in Nagpur City
Nagpur is the third largest city in the state of Maharashtra and ranks 13th in terms of population in India. Nagpur is a central part of India, with a population of more than 2.4 million (Census 2011 ). The average waste collection in Nagpur is 1119 TPD, out of which only 200 TPD is processed by Hanjer Biotech Energies Pvt. Ltd. This company produces 35–40 tonnes of compost daily, which is sold to Rashtriya Chemicals and Fertilizers (Times News Network 2017 ). A waste characterisation of Nagpur city was performed by CSIR-NEERI in all ten zones of the city. The sampling sites were decided based on different sources of wastes, viz. residential (slum and non-slum areas) and institutional areas, community bins, commercial areas, and disposal site. The household waste composition indicated a 77% organic fraction, 11.60% plastic, 7.66% paper, and 3.74% textiles and cardboard. However, the average value of all ten zones had a waste composition comprising 60% organic fraction, 15.50% plastic, 11.20% paper, 2.10% inerts, and the remaining 11% including wood, metal, glass, and other parts (Dutta 2017 ). The total waste dumped during the year 2016–2017 was estimated at approximately around 14,000 TPD by the Nagpur Municipal Corporation (NMC). Initially, the garbage was processed for bio-mining and then segregation, harrowing, and finally spraying of bio-cultures to speed up the degradation. This process proved effective in reducing the height of the piled waste to some extent. However, there is a need to market the produced compost. A recent massive fire and the unpleasant odour of the waste indicate that enormous challenges remain (Times News Network 2017 ).
The NMC also launched one project in June 2017 to deal with garden waste. This was a pilot project: they planned to include five gardens in the city and a women’s self-help group in the processing of biodegradable waste. This initiative was undertaken to reduce the burden of waste, in terms of transportation, as well as at the dumpsite. Provision was made to utilise the compost produced by any garden in the same garden (Dutta 2017 ).
4.4 The Scenario of Composting in Alappuzha and Thiruvananthapuram
Alappuzha and Thiruvananthapuram are cities located in the state of Kerala in the southern part of India. The populations of Alappuzha and Thiruvananthpuram 1,74,176 and 7,43,691, respectively (Census 2011 ). The Centre for Science and Environment, which is a not-for-profit public interest research and advocacy organisation based in New Delhi, assessed the performance of 20 cities for waste segregation at source, transportation, waste processing, and adoption of decentralised systems. In this assessment, these two cities won the four-leaf award and were ranked second in position, after Vengurla, which is a town in the Sindhudurg district of Maharashtra. Under the “Clean Home, Clean City” (Nirmal Bhavan, Nirmal Gram) initiative, the Alappuzha established a pipe composting system, an aerobic composting system, and biogas plants. The municipality set up fixed as well as portable biogas plants in both residential and public places. According to the renowned magazine Down to Earth , this pilot project soon was successful and benefited almost 40,000 households in 52 wards (Agarwal 2017 ). This progress of waste management was the result of a protest in 2012 in response to the shutting down of the dumping site at Sarvodayapuram, where garbage had been dumped for decades. This protest turned into a movement, and cameras were installed to catch people who litter. Alappuzha saved approximately 11 Lacs Rupees on transportation and liquefied petroleum gas bills. The city was recognised by United Nations Environmental Programme (UNEP), alongside four other anti-pollution cities across the world. The other four cities in the list were Osaka (Japan), Ljubljana (Slovenia), Penang (Malaysia), and Cajica (Colombia) (Agarwal 2017 ).
In Thiruvananthapuram, composting is being practised at the household level. Thiruvananthapuram consists of 100 wards, and each ward has 2500–3000 households (Bhardwaj 2017 ). The residents receive assistance on the technical element of composting. For composting at the source, local service providers put forward a three-layered bin and 30 l coco peat-based inoculum. The local service provider collects only the biodegradable waste. The technician will assist in the operations and also collects the compost if it is not required by the household and charges 200 Rupees monthly for providing the services (Bhardwaj 2017 ). The residents are also encouraged to use biodegradable products and to boycott non-biodegradable products through a programme called “Green Protocol”, which was launched by the state government of Kerala during the Onam celebrations, which is Kerala’s grand festival.
4.5 The Overall Scenario of Composting in India
Organic matter makes up more than 65% of MSW generated in India (NEERI Report 2012 ). If this organic fraction of MSW is processed by employing technologies like composting and anaerobic digestion, it can result in a reduction of 50% of the load on landfill sites. In India, only 10–12% of the total MSW is processed by means of composting, and the resulting compost possesses a poor nutrient value and mostly contains heavy metals like zinc, copper, lead, cadmium, lead, nickel, and chromium in higher concentrations as compared to the prescribed standard for compost (Hoornweg et al. 2000 and Waste to Energy Research Council 2012 ). This poor quality of compost is mainly due to the mixed nature of the waste (Hoornweg et al. 2000 ). Due to a lack of a continuous supply of feedstock to the compost plant and improper management, composting of biodegradable waste is not successful at large scale in India.
Similarly, improper segregation of waste, higher costs involved in the operation and maintenance of composting plants, and higher costs of manure than chemical fertiliser are major reasons for its non-viability in the Indian context (UNEP 2004 ). From a field survey, it was observed that many places in India face a failure of composting plants. For example, a windrow composting plant at Durgapur (300 TPD) and Kamarhati (200 TPD), West Bengal, was not able to achieve sales breakeven point due to issues with the waste characteristics; the plants are about to close. In the same state of West Bengal, the vermicompost plant at Chandannagar and Kalyani is performing well, but the vermicompost plants at Panihati (100 TPD), Bhadreshwar (20 TPD), and Khardah (30 TPD) are in poor shape because of the large variation in the waste characteristics and lack of supervision in the quality control of the product. The same situation applies to the vermicomposting plant (50 TPD) at Bidhannagar. The Kolkata Municipal Corporation is operating a 200 tonnes capacity windrow composting plant sporadically, at less than one-fourth of its capacity, due to the variation in waste characteristics. The compost plants at Mangalore (300 TPD) and Mysore (400 TPD) in the state of Karnataka and a 500 TPD compost plant situated at Nasik in the state of Maharashtra are also processing at lower plant capacity (Aich and Ghosh 2019 ).
5 Capacity Building Efforts: Strategies and Schemes Launched by the Government of India
Indian MSW management has good potential for composting as waste because it contains a significant amount of organic waste. A decentralised composting system is an appropriate option for India to efficiently run a composting unit. The Indian Government is also encouraging decentralised composting systems, since many environmentalists, policymakers, and researchers have advised that composting is the most feasible and effective waste management technique, in comparison to other techniques (Aich and Ghosh 2019 ). The government has also developed schemes for MSW capacity building in the country (Ministry of Chemicals and Fertilisers (MOC&F) 2017 ). Door-to-door waste collection and segregation at the source aid in the collection of waste that has similar characteristics and its disposal at the right place in an economical and efficient manner. A Market Development Assistance scheme has also been launched to enhance capacity building for the compost market. The Indian Government is encouraging all stages of capacity building for city compost. In the last few years, all countries throughout the globe have begun developing strategies for the management of solid waste in a sustainable manner. Composting, as a part of integrated solid waste management, is effective in almost all scenarios. In view of this, the Government of India has also framed different policies and strategies to encourage the practice of composting in India. Schemes like “Swachh Bharat Mission” promote the concept of cleaner and greener cities. Most of the challenges related to the efficient management of MSW in the country are stated in the CPHEEO ( 2016 ). The management of solid waste in the country is among the key factors in urban development, and for this reason, the Government of India has launched different schemes, including the Atal Mission for Rejuvenation and Urban Transformation (AMRUT), Jawaharlal Nehru National Urban Renewal Mission (JNNURM), Swachh Bharat Mission, and Smart Cities Mission. In these schemes, solid waste management is generally associated with the water supply system, storm drain system, and sewerage system. Regarding organic waste management, in particular, one recent report, “34th Report on Implementation of Policy on Promotion of City Compost”, was presented in the Parliament (Lok Sabha) on 10 April 2017. This report addressed one of the most serious problems associated with urbanisation. According to this report, the stated framework of City Compost is explained in the following section:
The “Swachh Bharat Mission” scheme is very closely associated with organic waste processing and its use as compost. Hence it can be considered as a rational part of this scheme.
The composting process helps in reducing the amount of waste at landfill sites.
Composting also prevents GHG emissions and harmful toxic materials from leaching into groundwater.
The organic carbon constituent of compost is useful in maintaining and improving soil fertility.
According to the Ministry of Urban Development, the entire potential of city compost plant in India is 0.71 MT per year, while the current production is 0.15 MT per year.
In the view of this, the MOC&F of the Government of India released a notification in Parliament (Lok Sabha), dated 10 February 2016, to promote city compost schemes (MOC&F 2017 ). The key features of the scheme are as follows:
An amount of 1500/− Rupees will be provided per tonnes of city compost in the form of marketing development, which in turn will encourage the manufacturing and marketing of the city compost.
At the initial stage, the existing fertiliser manufacturer will be promoted by the marketing of city compost. Meanwhile, other marketing entities marked by concerned state governments may also be involved, with the approval of the Department of Fertilisers.
The manufacturer involved in the promotion and sale of city compost may get a subsidy as per the decision of the Department of Fertilisers.
A fertiliser manufacturer can co-market city compost with chemical fertilisers through their network in the market.
Companies will adopt the village to encourage city compost utilisation.
The government and public sectors in India will also promote the use of city compost for their gardens and horticulture, to the greatest possible extent.
Farmers should be educated about the benefits of city compost through information, education, and communication campaign conducted by the Department of Agriculture, Cooperation, and Farmers’ Welfare. Agricultural universities will also participate in the same.
The Ministry of Urban Development will look after installations of the compost plants across the state.
A Bureau of Indian Standards (BIS) or eco-mark will be developed with the association of BIS to ensure good quality of compost and so its acceptance among farmers.
Government bodies like the Department of Agriculture and Fertilisers and the Ministry of Urban Development will be involved in monitoring to provide information on the availability of city compost. This mechanism will help compost manufacturers and fertiliser marketing concerning coordination issues.
Expenditure on market development assistance will be met from the budget provision for the Department of Fertilisers.
As a result of this notification, fertiliser companies have adopted 100 villages to promote the city compost. After the implementation of this policy, the production of compost plants is expected to increase, and the target is the processing of 100% of MSW. Significant results are expected to be seen in a year (MOC&F 2017 ). Decentralised composting units are a feasible option, but skilled manpower is required in good strength. The Indian Government is helping right from waste pickers to farmers with appropriate schemes for the efficient management of organic waste in a sustainable manner.
The Indian Government is also taking steps towards spreading awareness among citizens and by providing e-learning for MSW practices. These courses involve case studies to explain the appropriate options for particular situations. Specialised courses on composting are included in the subcategory of the 200 series (205, 206, 214, 217, 230, 235, and 252) (CPHEEO 2016 ). This course has been designed to systematically enhance capacity building on composting in India. These courses comprise several case studies of Indian cities, such as Vijayawada, Coimbatore, Bengaluru, Thiruvananthapuram, and Kochi. Apart from the case studies, these courses offer training on choosing suitable composting facilities (CPHEEO 2016 ).
6 Conclusion and Recommendations
Composting has been practised in India for a long time. However, the extent of its use is surging with time and need. At present, waste management has become a major challenge due to the unscientific practices of waste management observed in the past few decades. Composting technology can be successful or may fail: this depends on its planning and execution. Decentralised composting is burgeoning in India, as the management of smaller units is comparatively easier than bigger units, and the installation cost also affects the financial sustainability of the operational plant. Implementing composting plants of greater capacity generally requires huge investments, and recovering the invested amounts becomes difficult; thus, the losses incurred may lead to the closure of the plants. As discussed earlier, the installation of decentralised smaller composting units is effective at the ground level (MHUA 2018 ).
One of the major factors in the sustenance of composting plants is community participation. The active participation of the general masses is extremely important; if waste is segregated at the source itself, then the management of waste can be done most effectively. Earlier, the Indian population was negligent towards waste segregation; however, changes in behaviour started when Indians started experiencing the unpleasant views, odours, and health issues that arise due to growing volumes of MSW left unmanaged. Moreover, awareness of the health risks related to unprofessional MSW management has increased. Cities like Alappuzha, Thiruvananthapuram, Mysuru, Panjim, and Panchgani are progressing towards the concept of zero waste discharge and becoming self-sustainable cities. In mega metro cities, adopting composting technology for effective waste management is still a major issue, due to behemoth volumes of waste generation, and in big cities, composting is still a big issue as the amount of waste generated is too huge to manage.
Busy lifestyles, coupled with higher living standards, in the urban parts of the country have also led to negligence regarding the management of waste. However, the critical steps taken by the government to manage generated wastes and put to better use have also resulted in a change in the perspective of the general population. It has been observed that the population is becoming aware of problems associated with effective waste management and is becoming more conscious regarding the effectual management of waste. People are sensitised about this topic, and they are trying to involve themselves more in waste management activities.
Adopting composting techniques for processing organic waste has shown a fair reduction in emissions, as well as in the cost involved in different waste management techniques. Cities with successful operation composting facilities are inspiring other cities to adopt the same, thus resulting in a reduction in their investment and emissions. Apart from these benefits, the general population is also experiencing health benefits, which are also extremely important. If the metro cities plan to install large-scale composting units, precise planning for the recovery of cost should be done in advance by forming various market strategies. It will always prove beneficial to install and operate a pilot-scale composting unit rather than directly going for large-scale composting plants. A reconnaissance study of the concerned region, along with the characteristics of the waste and the acceptance of the technology, should be carried out before the installation of the composting plants. Different urban parts of the country are gradually coming forward to join the initiative for sustainable waste management in India. The government is also encouraging them to manage their waste by providing technical assistance, subsidies for plant installation, and a compost market. The government schemes and strategies are also playing a paramount role in successful compost plant operation.
As described in previous sections, several schemes supporting the composting of organic waste generated from the urban regions of the country have been launched and promoted by the Government of India. Despite all the policies and schemes framed by the government, their implementation on the ground level is still a major challenge. From experience, it has been found that the ruling government has tried to implicate all the perspectives of a composting technique for making it feasible. Different states in the country have different perspectives on and different visions of composting. Sates which have been practising composting for a few years have a commercial approach, while states in the country which are planning to adopt composting to manage their waste have a sustainable approach, ultimately resulting in sustainable development. The efforts taken by the government to promote composting technology should be appreciated, and the policies should be strictly followed to ensure their successful implementation. It is the fundamental duty of every citizen to consider their country as their own home and to take care to keep it green and clean .
Adak, B. (2018). North Delhi to get green waste compost plant. India Today . Available at: https://www.indiatoday.in/mail-today/story/north-delhi-to-get-green-waste-compost-plant-1283362-2018-07-12 . Accessed 3 Oct 2018.
Agarwal, R. (2017). Alappuzha gets recognised by UNEP for its solid waste management practices. DowntoEarth . Available at: https://www.downtoearth.org.in/news/alappuzha-gets-recognized-by-unep-for-its-solid-waste-management-practices-59258 . Accessed 3 Oct 2018.
Aich, A., & Ghosh, S. K. (2019). Conceptual framework for municipal solid waste processing and disposal system in India. In Waste management and resource efficiency (pp. 91–107). Singapore: Springer.
Chapter Google Scholar
Basu, T. (2014). New law of the land: Compost or perish, The Hindu Business Line . Available at: https://www.thehindubusinessline.com/news/variety/new-law-of-the-land-compost-or-perish/article23030221.ece . Accessed 2 Oct 2018.
Belyaeva, O. N., & Haynes, R. J. (2009). Chemical, microbial and physical properties of manufactured soils produced by co-composting municipal green waste with coal fly ash. Bioresource Technology, 100 , 5203–5209.
Article CAS Google Scholar
Bhardwaj, D. (2017). With the help of residents, this is how Trivandrum is composting its own waste. The Better India .
Google Scholar
Bhattacharya, S. (2016). A tiny town in West Bengal is turning waste into piles of wealth. Hindustan Times .
Census. (2011). Primary census abstracts . Registrar General of India, Ministry of Home Affairs, Government of India.
CPCB. (2013a). CPCB annual report 2011–12. Ministry of environment . Forest and Climate Change, Government of India.
CPCB. (2013b). Status report on municipal solid waste management 1–13 . Ministry of Environment, Forest and Climate Change, Government of India.
CPHEEO. (2016). Swachh Bharat mission municipal solid waste management manual . Central Public Health & Environmental Engineering Organisation. Ministry of Housing and Urban Affairs, Government of India.
Desai, K. (2018). How these temples give a new life to old flowers. The Times of India . Available at: https://timesofindia.indiatimes.com/india/how-these-temples-give-a-new-life-to-old-flowers/articleshow/65762167.cms . Accessed 3 Oct 2018.
Diener, S., Zurbrügg, C., & Tockner, K. (2015). Bioaccumulation of heavy metals in the black soldier fly, Hermetia illucens and effects on its life cycle. Journal of Insects as Food and Feed, 1 , 261–270.
Article Google Scholar
Dinesh, M. S., Geetha, K. S., Venugopalan, V., Kale, R. D., & Murthy, V. K. (2010). Ecofriendly treatment of biomedical wastes using epigeic earthworms. Journal of Indian Society of Hospital Waste Management, 9 (1).
Dutta, S., (2017). From composting garden waste to treating 200 tonnes of waste daily, Nagpur’s techniques show its all round efforts in improving waste management . Swachh India. Accessed 3 Oct 2018.
Dutta, S. (2018). Kolkata municipal corporation’s waste management practices come under state pollution control board’s scanner. Swachh India. Accessed 2 Oct 2018.
Fang, M., Wong, J. W. C., Ma, K. K., & Wong, M. H. (1999). Composting of sewage sludge and coal fly ash: Nutrient transformations. Bioresource Technology, 67 , 19–24.
Gajalakshmi, S., & Abbasi, S. A. (2008). Solid waste management by composting: State of the art. Critical Reviews in Environmental Science and Technology., 38 (5), 311–400.
Halder, R. (2017). Five colonies of Central Delhi to turn zero-waste by 2018. Hindustan Times .
Hoornweg, D., & Bhada-Tata, P. (2012). What a waste: A global review of solid waste management. ANNEX J: MSW generation by country — Current data and projections for 2025 (Urban Development Series). Washington, DC: The World Bank.
Hoornweg, D., Thomas, L., & Otten, L. (2000). Composting and its applicability in developing countries (Working Paper Series 8). Washington DC: The World Bank.
Hubbe, M. A., Nazhad, M., & Sánchez, C. (2010). Composting as a way to convert cellulosic biomass and organic waste into high-value soil amendments: A review. BioResources, 5 , 2808–2854.
Jouraiphy, A., Amir, S., El Gharous, M., Revel, J. C., & Hafidi, M. (2005). Chemical and spectroscopic analysis of organic matter transformation during composting of sewage sludge and green plant waste. International Biodeterioration and Biodegradation, 56 , 101–108.
Karthigeyan, M., & Alagesan, P. (2011). Millipede composting: A novel method for organic waste recycling. Recent Research in Science and Technology, 3 (9), 62–67.
Kumar, S. (2011). Composting of municipal solid waste. Critical Reviews in Biotechnology, 31 , 112–136.
Kumar, S., Bhattacharyya, J. K., Vaidya, A. N., Chakrabarti, T., Devotta, S., & Akolkar, A. B. (2009). Assessment of the status of municipal solid waste management in metro cities, state capitals, class I cities, and class II towns in India: An insight. Waste Management, 29 , 883–895.
Kumar, S., Negi, S., Mandpe, A., Singh, R. V., & Hussain, A. (2018). Rapid composting techniques in Indian context and utilization of black soldier fly for enhanced decomposition of biodegradable wastes: A comprehensive review. Journal of Environmental Management, 227 , 189–199.
Lou, X. F., & Nair, J. (2009). The impact of landfilling and composting on greenhouse gas emissions: A review. Bioresource Technology, 100 , 3792–3798.
Manyapu, V., Mandpe, A., & Kumar, S. (2017). Synergistic effect of fly ash in in-vessel composting of biomass and kitchen waste. Bioresource Technology, 251 , 114–120.
Ministry of Housing and Urban Affairs (MHUA). (2018). Urban advisory on on-site and decentralized composting of municipal organic waste . Swachh Bharat Mission.
MOC&F. (2017). Standing committee on chemicals and fertilizers (2014–15) .
Monson, C. C., & Murugappan, A. (2010). Developing optimal combination of bulking agents in an in-vessel composting of vegetable waste. E-Journal of Chemistry, 7 , 93–100.
Mudgal, S., (2015). Make wealth from waste, DowntoEarth . Available at: https://www.downtoearth.org.in/coverage/waste/make-wealth-from-waste-47164 . Accessed 3 Oct 2018.
National Environmental Engineering Research Institute (NEERI) Report. (2012). Optimisation of organic waste to energy systems in India .
Planning Commission. (2014). Report of the task force on waste to energy: Volume I. Task Force Waste to Energy, I , 1–178.
Ramachandra, T. V., Bharath, H. A., Kulkarni, G., & Han, S. S. (2018). Municipal solid waste: Generation, composition and GHG emissions in Bangalore, India. Renewable and Sustainable Energy Reviews, 82 , 1122–1136.
Rao, P.V. (2016). A small town in West Bengal helped Kolkata win a global award for waste management. The Better India .
Sharholy, M., Ahmad, K., Mahmood, G., & Trivedi, R. C. (2008). Municipal solid waste management in Indian cities: A review. Waste Management, 28 , 459–467.
Times News Network. (2013). Only one-tenth of waste recycled in Kolkata: Study. The Times of India .
Times News Network. (2017). Nagpur Municipal Corporation to convert garden waste into compost. The Times of India .
Tomić, T., & Schneider, D. R. (2017). Municipal solid waste system analysis through energy consumption and return approach. Journal of Environmental Management, 203 , 973–987.
UNEP. (2004). State of waste management in South East Asia..
Vandergheynst, J. (2009). Compost processing and its relationship to compost quality . Biological and Agricultural Engineering, UC Davis.
Varma, S. V., & Kalamdhad, A. S. (2013). Composting of Municipal Solid Waste (MSW) mixed with cattle manure. International Journal of Environmental Sciences, 3 (6), 2068–2079.
Waste to Energy Research Council. (2012). Sustainable solid waste management in India . Earth Engineering Centre Columbia.
Wei, Y., Li, J., Shi, D., Liu, G., Zhao, Y., & Shimaoka, T. (2017). Environmental challenges impeding the composting of biodegradable municipal solid waste: A critical review. Resources, Conservation and Recycling, 122 , 51–65.
Download references
Author information
Authors and affiliations.
Council of Scientific & Industrial Research (CSIR)-National Environmental Engineering and Research Institute (CSIR-NEERI), Nagpur, India
Ashootosh Mandpe, Sweta Kumari & Sunil Kumar
You can also search for this author in PubMed Google Scholar
Editor information
Editors and affiliations.
United Nations University (UNU-FLORES), Dresden, Sachsen, Germany
Hiroshan Hettiarachchi
Serena Caucci
Thünen Institute of Forest Systems, Eberswalde, Germany
Kai Schwärzel
Rights and permissions
The opinions expressed in this chapter are those of the author(s) and do not necessarily reflect the views of the United Nations University Institute for Integrated Management of Material Fluxes and of Resources (UNU-FLORES), its Board of Directors, or the countries they represent
Open Access This chapter is licensed under the terms of the Creative Commons Attribution 3.0 IGO license (https://creativecommons.org/licenses/by/3.0/igo/), which permits use, sharing, adaptation, distribution and reproduction in any medium or format, as long as you give appropriate credit to the United Nations University Institute for Integrated Management of Material Fluxes and of Resources (UNU-FLORES), provide a link to the Creative Commons license and indicate if changes were made.
Any dispute related to the use of the works of the United Nations University Institute for Integrated Management of Material Fluxes and of Resources (UNU-FLORES) that cannot be settled amicably shall be submitted to arbitration pursuant to the UNCITRAL rules. The use of the United Nations University Institute for Integrated Management of Material Fluxes and of Resources (UNU-FLORES)'s name for any purpose other than for attribution, and the use of the UNU-FLORES's logo, shall be subject to a separate written license agreement between the UNU-FLORES and the user and is not authorized as part of this CC-IGO license. Note that the link provided above includes additional terms and conditions of the license.
The images or other third party material in this chapter are included in the chapter's Creative Commons license, unless indicated otherwise in a credit line to the material. If material is not included in the chapter's Creative Commons license and your intended use is not permitted by statutory regulation or exceeds the permitted use, you will need to obtain permission directly from the copyright holder.
Reprints and permissions
Copyright information
© 2020 The Author(s)
About this chapter
Mandpe, A., Kumari, S., Kumar, S. (2020). Composting: A Sustainable Route for Processing of Biodegradable Waste in India. In: Hettiarachchi, H., Caucci, S., Schwärzel, K. (eds) Organic Waste Composting through Nexus Thinking. Springer, Cham. https://doi.org/10.1007/978-3-030-36283-6_3
Download citation
DOI : https://doi.org/10.1007/978-3-030-36283-6_3
Published : 24 November 2020
Publisher Name : Springer, Cham
Print ISBN : 978-3-030-36282-9
Online ISBN : 978-3-030-36283-6
eBook Packages : Biomedical and Life Sciences Biomedical and Life Sciences (R0)
Share this chapter
Anyone you share the following link with will be able to read this content:
Sorry, a shareable link is not currently available for this article.
Provided by the Springer Nature SharedIt content-sharing initiative
- Publish with us
Policies and ethics
- Find a journal
- Track your research
- Skip to main content
- Keyboard shortcuts for audio player

- Dear Life Kit
- Life Skills

- LISTEN & FOLLOW
- Apple Podcasts
- Amazon Music
Your support helps make our show possible and unlocks access to our sponsor-free feed.
Composting can help fight climate change. Get started in 5 easy steps
Julia Simon
Credit: Becky Harlan/NPR
This story was originally published on April 8, 2020, and has been updated.
Climate change is affecting our food, and our food is affecting the climate. NPR is dedicating a week to stories and conversations about the search for solutions .
About 8% of planet-heating emissions come from wasted food , and about 30% to 40% of food gets wasted in the United States.
But as much as you're meal planning and reducing your food waste , there are certain things you're just not going to eat — like banana peels, or, if you're me, a frightening amount of pineapple tops.
Keeping food out of landfills can help fight climate change . And, luckily, there's an easy solution for your home food waste: Composting!
It doesn't matter if you're in a suburban home or a tiny apartment. This guide will help you turn your food waste into beautiful earthy compost in five simple steps.
1. Select your food scraps

Start with fruits and veggies — the skin of a sweet potato, the top of your strawberry. Also tea bags, coffee grounds, eggshells, old flowers — even human hair!
Meat and dairy products, though, are asking for trouble. Leonard Diggs is the director of operations at the Pie Ranch Farm in Pescadero, California. He says you gotta ask yourself, "Do you attract rodents? Do you attract animals to your pile? Meat products are likely to do that."
Other things that may attract pests? Cooked food, oily things, buttery things and bones.
Also important to note that some products say "compostable" on them — like "compostable bags" and "compostable wipes." Those are compostable in industrial facilities, but they don't really work for home composting.
2. Store those food scraps

How To Reduce Food Waste
When you're composting, your kitchen scraps should be part of a deliberate layering process to speed up decomposition. There's a method for adding them to the pile ( see step 4! ), so you'll need to store them in a container so you can add them bit by bit.
"It doesn't have to be, you know, all the things that you find online that are really cute little ceramic containers," says Diggs. He says it "can just be an old milk carton. When you make the first chop of the butt of that asparagus, boom, it could go right in there."
Also, you can store the food scraps in a bag in your freezer or the back of the fridge. That's an easy way to avoid odors and insects in your kitchen.
3. Choose a place to make your compost
Worms make great pets, and other reasons to compost at home.
For this step, you gotta think about the space you're currently living in.
If you don't have a backyard and still want a traditional composting experience you can take your food scraps to a compost pile that you share with neighbors or at a community garden.
If you want to break down your food scraps in your own apartment, there are still options. Jeffrey Neal , founder and chief executive of Loop Closing , a composting business in Washington, D.C., is a big fan of worms . He says you don't need a big container for " vermicomposting " — a 5 gallon box will do. Or you can go bigger.
"There are times when I made [my worm box] an ottoman so I could relax with my feet up on them! You can use it like a piece of furniture."
Another small space idea, Neal says, is fermenting your food scraps with a Japanese method called Bokashi . "All you need is a container you can seal and Bokashi mix, a colony of bacteria on grain." (Here's some more info on how to use worms and Bokashi.)
Of course, it's totally fine if you want to give your food scraps to someone else to make compost. Some municipalities will pick up your food scraps from your home. You can also ask your local grocery stores, restaurants or farmers markets to see if they have programs to take food scraps.
If you do have some outdoor space, your compost bin doesn't have to be complicated. "I think keeping it simple," Diggs says. An old trash bin, an old wooden chest — just work with what you have available.
You can also buy a bin online or Diggs says, "You could just create the pile naked!" Basically you can just have a heap of compost — but don't put it up against a wall as it could stain it.
4. Make the compost mix

In the world of composting you're inevitably gonna hear about "the greens and browns" — the two main ingredients for your mix.
"Greens" are typically food scraps, like fruit and vegetable peelings, coffee grounds, or, if you have a yard, grass clippings. These add nitrogen — a crucial element for microbial growth. Microorganisms are the true heroes of this process, they do the heavy lifting of decomposition.
"Browns" are more carbon rich — think egg cartons, newspapers, dried leaves, and pine needles. It helps to shred up the paper products before putting them in your pile.
A good thing to remember is that green materials are typically wet, and brown materials are typically dry. When you're layering, you want the dry browns on the bottom with the wet greens on the top.

This Is A Good Time To Start A Garden. Here's How
Diggs says the browns are key because they allow water to flow, and air to flow, something called aeration. That will make sure microorganisms can do their job. "If one hundred percent of it is water, then nothing is going on. The microorganisms can't work. You got this soggy, smelly pile," Diggs says, "So drainage makes a difference."
A helpful analogy is to think of tending to your compost like tending a fire. Just as in a fire you need to structure the wood to get the air going, in compost you have to do a similar thing, adding spaces to give oxygen to those heroic microbes.
And it really is layering — browns then greens, browns then greens. The number of layers depends on your space and your amount of food scraps, but try to keep the layers to an inch or two. You can also put a little bit of browns on the very top to keep away flies and odors.
As for the ratio of "browns" to "greens," you often hear three or four parts of browns to one part greens. Sometimes two to one. Ultimately you always want more browns than greens — again, gotta have the dry to sop up the wet.
5. Wait and aerate

How To Talk To Kids About Climate Change
How long do you have to wait for decomposition? "If it's hot, you could get there in two months pretty easy, " Diggs says, "If it's cold made, you could be there in six months. And for every component to break down, it might be a year."
To keep things moving, you'll want to turn or rotate the pile, perhaps with a stick or spade. Remember the fire analogy — you gotta make sure the air is flowing, that it's wet but not too soggy.
As for how much you turn it, you'll probably turn it less if you have the right ratio of greens to browns. Diggs says when you start out you might be turning the compost once every seven to 10 days.
Typically the more compost you have, the faster it will go.
Neal says in the end "the nose knows" when your compost is ready. "Bad compost smells, well, bad," he says, "It's like what a smelly trash can or dumpster smells like ... Basically, it smells like a landfill."
If it smells bad, it probably means it's not decomposing — maybe your pile might be too wet or you might need to readjust your ratios of greens and browns.
Diggs says he loves smelling finished compost,"You know, it just smells so ... Oh, gosh. Woody, earthy, but also a sweet smell. Or sometimes a sour smell. And the feel! How fluffy it is!"
When you've got that fluffy, earthy compost, put it in your garden, or in a plant on your windowsill. Or you can donate to your local community garden — just be sure to text ahead!
Of course composting takes patience — you might run into unexpected things. We don't want you to give up so here are some more resources below.
The Texas A&M AgriLife Extension has an excellent " compost trouble-shooting guide ." For example, it has suggestions of what to do if the pile has insects or is too wet.
- Jeffrey Neal from Loop Closing has compiled resources for those looking to try worm composting or Bokashi .
- Oregon State has a comprehensive guide for composting and "vermicomposting" — using a worm composter to break down organic materials.
- Cornell University's Waste Management Institute has a more detailed guide to composting and "greens" and "browns," plus a lot more resources on their website.
We'd love to hear from you. If you have a good life hack, leave us a voicemail at 202-216-9823 or email us at [email protected]. Your tip could appear in an upcoming episode.
Want more Life Kit? Subscribe to our newsletter .
The audio portion of this story was produced by Audrey Nguyen.

Roundup: Your Tips To Fight Food Waste
- Life Kit: Life Skills
Information
- Author Services
Initiatives
You are accessing a machine-readable page. In order to be human-readable, please install an RSS reader.
All articles published by MDPI are made immediately available worldwide under an open access license. No special permission is required to reuse all or part of the article published by MDPI, including figures and tables. For articles published under an open access Creative Common CC BY license, any part of the article may be reused without permission provided that the original article is clearly cited. For more information, please refer to https://www.mdpi.com/openaccess .
Feature papers represent the most advanced research with significant potential for high impact in the field. A Feature Paper should be a substantial original Article that involves several techniques or approaches, provides an outlook for future research directions and describes possible research applications.
Feature papers are submitted upon individual invitation or recommendation by the scientific editors and must receive positive feedback from the reviewers.
Editor’s Choice articles are based on recommendations by the scientific editors of MDPI journals from around the world. Editors select a small number of articles recently published in the journal that they believe will be particularly interesting to readers, or important in the respective research area. The aim is to provide a snapshot of some of the most exciting work published in the various research areas of the journal.
Original Submission Date Received: .
- Active Journals
- Find a Journal
- Proceedings Series
- For Authors
- For Reviewers
- For Editors
- For Librarians
- For Publishers
- For Societies
- For Conference Organizers
- Open Access Policy
- Institutional Open Access Program
- Special Issues Guidelines
- Editorial Process
- Research and Publication Ethics
- Article Processing Charges
- Testimonials
- Preprints.org
- SciProfiles
- Encyclopedia
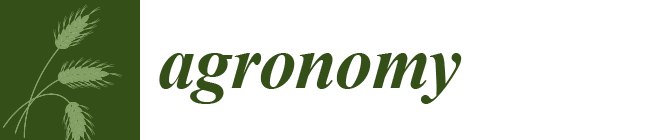
Article Menu
- Subscribe SciFeed
- Recommended Articles
- Google Scholar
- on Google Scholar
- Table of Contents
Find support for a specific problem in the support section of our website.
Please let us know what you think of our products and services.
Visit our dedicated information section to learn more about MDPI.
JSmol Viewer
Recycling of organic wastes through composting: process performance and compost application in agriculture.
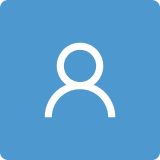
1. Introduction
2. composting process, 2.1. process parameters, 2.2. composting stages, 2.3. stability and maturity of compost, 3. application of compost in agriculture, 3.1. the ideal form of nutrients in the compost, 3.2. implication of maturity and stability on the compost quality in agriculture, 3.3. effects of compost on biological and enzymatic activities in soils, 3.4. effects of compost on soil physical properties, 3.4.1. soil aggregate and stability, 3.4.2. bulk density, 3.4.3. infiltration rate and water-holding capacity, 3.5. effect of compost on chemical properties of soil, 3.5.1. enhancement of nutrient level, 3.5.2. cation exchange capacity (cec) and ph value, 3.6. effects of compost on crop productivity and yield, 3.7. effects of compost on plant pathogens and diseases, 4. application of compost as growing media, 5. compost application as bioremediation agent for contaminated soil, 6. potential risks of compost application in agriculture, 7. conclusions, author contributions, acknowledgments, conflicts of interest.
- Chen, P.; Xie, Q.; Addy, M.; Zhou, W.; Liu, Y.; Wang, Y.; Cheng, Y.; Yanling, C.; Ruan, R. Utilization of municipal solid and liquid wastes for bioenergy and bioproducts production. Bioresour. Technol. 2016 , 215 , 163–172. [ Google Scholar ] [ CrossRef ] [ PubMed ] [ Green Version ]
- Maina, S.; Kachrimanidou, V.; Koutinas, A. A roadmap towards a circular and sustainable bioeconomy through waste valorization. Curr. Opin. Green Sustain. Chem. 2017 , 8 , 18–23. [ Google Scholar ] [ CrossRef ]
- Soobhany, N. Insight into the recovery of nutrients from organic solid waste through biochemical conversion processes for fertilizer production: A review. J. Clean. Prod. 2019 , 241 , 118413. [ Google Scholar ] [ CrossRef ]
- The World Bank. Solid Waste Management. 2019. Available online: https://www.worldbank.org/en/topic/urbandevelopment/brief/solid-waste-management (accessed on 1 March 2020).
- Oliveira, L.S.; Oliveira, D.S.; Bezerra, B.S.; Pereira, B.S.; Battistelle, R.A.G. Environmental analysis of organic waste treatment focusing on composting scenarios. J. Clean. Prod. 2017 , 155 , 229–237. [ Google Scholar ] [ CrossRef ] [ Green Version ]
- EC. Circular Economy—Implementation of the Circular Economy Action Plan. European Commission. 2018. Available online: http://ec.europa.eu/environment/circular-economy/index (accessed on 1 March 2020).
- EC. Proposal for a Regulation on the Making Available on the Market of CE Marked Products Fertilising and Amending Regulations (EC) No 1069/2009 and (EC) No 1107/2009. COM (2016) 157 Final 2016/00084 (COD). European Commission. 2016. Available online: https://ec.europa.eu/transparency/regdoc/rep/1/2016/EN/1-2016-157-EN-F1-1.PDF (accessed on 1 March 2020).
- Ţurcanu, M. Review of the Fertilizing Products Regulation. European Parliament. 2018. Available online: http://www.europarl.europa.eu/legislative-train/theme-new-boost-for-jobs-growthand-investment/file-review-of-the-fertilising-products-regulation (accessed on 1 March 2020).
- Ghosh, P.K.; Ramesh, P.; Bandyopadhyay, K.K.; Tripathi, A.K.; Hati, K.M.; Mishra, A.K.; Acharya, C.L. Comparative effectiveness of cattle manure, poultry manure, phospho-compost and fertilizer-NPK on the cropping systems in vertisols of semi-arid tropics; crop yield and system performance. Bioresour. Technol. 2004 , 95 , 77–83. [ Google Scholar ]
- Meng, L.; Li, W.; Zhang, S.; Wu, C.; Lv, L. Feasibility of co-composting of sewage sludge, spent mushroom substrate and wheat straw. Bioresour. Technol. 2017 , 226 , 39–45. [ Google Scholar ] [ CrossRef ]
- Zhang, L.; Sun, X. Improving green waste composting by addition of sugarcane bagasse and exhausted grape marc. Bioresour. Technol. 2016 , 218 , 335–343. [ Google Scholar ] [ CrossRef ]
- Malińska, K.; Golańska, M.; Caceres, R.; Rorat, A.; Weisser, P.; Ślęzak, E. Biochar amendment for integrated composting and vermicomposting of sewage sludge—The effect of biochar on the activity of Eisenia fetida and the obtained vermicompost. Bioresour. Technol. 2017 , 225 , 206–214. [ Google Scholar ] [ CrossRef ]
- Wang, Q.; Wang, Z.; Awasthi, M.K.; Jiang, Y.; Li, R.; Ren, X.; Zhao, J.; Shen, F.; Wang, M.; Zhang, Z. Evaluation of medical stone amendment for the reduction of nitrogen loss and bioavailability of heavy metals during pig manure composting. Bioresour. Technol. 2016 , 220 , 297–304. [ Google Scholar ] [ CrossRef ]
- Garg, V.; Kaushik, P.; Dilbaghi, N. Vermiconversion of wastewater sludge from textile mill mixed with anaerobically digested biogas plant slurry employing Eisenia foetida. Ecotoxicol. Environ. Saf. 2006 , 65 , 412–419. [ Google Scholar ] [ CrossRef ]
- Papafilippaki, A.; Paranychianakis, N.V.; Nikolaidis, N.P. Effects of soil type and municipal solid waste compost as soil amendment on Cichorium spinosum (spiny chicory) growth. Sci. Hortic. 2015 , 195 , 195–205. [ Google Scholar ] [ CrossRef ]
- Sanasam, S.D.; Talukdar, N.C. Quality Compost Production from Municipality Biowaste in Mix with Rice Straw, Cow Dung, and Earthworm Eisenia fetida. Compos. Sci. Util. 2017 , 25 , 141–151. [ Google Scholar ] [ CrossRef ]
- Edgerton, M.D. Increasing Crop Productivity to Meet Global Needs for Feed, Food, and Fuel. Plant Physiol. 2009 , 149 , 7–13. [ Google Scholar ] [ CrossRef ] [ PubMed ] [ Green Version ]
- Zhao, B.; O’Connor, D.; Zhang, J.; Peng, T.; Shen, Z.; Tsang, D.C.; Hou, D. Effect of pyrolysis temperature, heating rate, and residence time on rapeseed stem derived biochar. J. Clean. Prod. 2018 , 174 , 977–987. [ Google Scholar ] [ CrossRef ]
- O’Connor, D.; Peng, T.; Zhang, J.; Tsang, D.C.; Alessi, D.S.; Shen, Z.; Bolan, N.; Hou, D. Biochar application for the remediation of heavy metal polluted land: A review of in situ field trials. Sci. Total Environ. 2018 , 619 , 815–826. [ Google Scholar ] [ CrossRef ] [ PubMed ]
- Aye, L.; Widjaya, E. Environmental and economic analyses of waste disposal options for traditional markets in Indonesia. Waste Manag. 2006 , 26 , 1180–1191. [ Google Scholar ] [ CrossRef ] [ PubMed ]
- Elagroudy, S.; Elkady, T.; Ghobrial, F. Comparative Cost Benefit Analysis of Different Solid Waste Management Scenarios in Basrah, Iraq. J. Environ. Prot. 2011 , 2 , 555–563. [ Google Scholar ] [ CrossRef ] [ Green Version ]
- Kim, M.H.; Song, Y.-E.; Song, H.-B.; Kim, J.-W.; Hwang, S.-J. Evaluation of food waste disposal options by LCC analysis from the perspective of global warming: Jungnang case, South Korea. Waste Manag. 2011 , 31 , 2112–2120. [ Google Scholar ] [ CrossRef ]
- Ju, X.-T.; Xing, G.-X.; Chen, X.-P.; Zhang, S.-L.; Zhang, L.-J.; Liu, X.-J.; Cui, Z.-L.; Yin, B.; Christie, P.; Zhu, Z.-L.; et al. Reducing environmental risk by improving N management in intensive Chinese agricultural systems. Proc. Natl. Acad. Sci. USA 2009 , 106 , 3041–3046. [ Google Scholar ] [ CrossRef ] [ Green Version ]
- Gai, X.; Liu, H.; Liu, J.; Zhai, L.; Wang, H.; Yang, B.; Ren, T.; Wu, S.; Lei, Q. Contrasting impacts of long-term application of manure and crop straw on residual nitrate-N along the soil profile in the North China Plain. Sci. Total Environ. 2019 , 650 , 2251–2259. [ Google Scholar ] [ CrossRef ]
- Choudhary, M.; Panday, S.C.; Meena, V.S.; Singh, S.; Yadav, R.P.; Mahanta, D.; Mondal, T.; Mishra, P.K.; Bisht, J.K.; Pattanayak, A. Long-term effects of organic manure and inorganic fertilization on sustainability and chemical soil quality indicators of soybean-wheat cropping system in the Indian mid-imalayas. Agric. Ecosyst. Environ. 2018 , 257 , 38–46. [ Google Scholar ]
- Kulikowska, D.; Gusiatin, Z.M.; Bułkowska, K.; Klik, B. Feasibility of using humic substances from compost to remove heavy metals (Cd, Cu, Ni, Pb, Zn) from contaminated soil aged for different periods of time. J. Hazard. Mater. 2015 , 300 , 882–891. [ Google Scholar ] [ CrossRef ] [ PubMed ]
- Zhao, S.; Shang, X.; Duo, L.A. Accumulation and spatial distribution of Cd, Cr, and Pb in mulberry from municipal solid waste compost following application of EDTA and (NH4)2SO4. Environ. Sci. Pollut. Res. 2012 , 20 , 967–975. [ Google Scholar ] [ CrossRef ]
- Głąb, T.; Żabiński, A.; Sadowska, U.; Gondek, K.; Kopeć, M.; Mierzwa–Hersztek, M.; Tabor, S. Effects of co-composted maize, sewage sludge, and biochar mixtures on hydrological and physical qualities of sandy soil. Geoderma 2018 , 315 , 27–35. [ Google Scholar ] [ CrossRef ]
- Weber, J.; Kocowicz, A.; Bekier, J.; Jamroz, E.; Tyszka, R.; Debicka, M.; Parylak, D.; Kordas, L. The effect of a sandy soil amendment with municipal solid waste (MSW) compost on nitrogen uptake efficiency by plants. Eur. J. Agron. 2014 , 54 , 54–60. [ Google Scholar ] [ CrossRef ]
- Haug, R.T. The Practical Handbook of Compost Engineering ; Lewis Publisher: Boca Raton, FL, USA, 1993. [ Google Scholar ]
- Roman, P.; Martinez, M.M.; Pantoja, A. Farmer’s Compost Handbook: Experiences in Latin America. Composting parameters and compost quality: A literature review. Org. Agric. 2015 , 8 , 141–158. [ Google Scholar ]
- Awasthi, M.K.; Selvam, A.; Lai, K.M.; Wong, J.W. Critical evaluation of post-consumption food waste composting employing thermophilic bacterial consortium. Bioresour. Technol. 2017 , 245 , 665–672. [ Google Scholar ] [ CrossRef ]
- Bertoldi, M.; de Vallini, G.; Pera, A. The Biology of Composting: A review. Waste Manag. Res. 1983 , 1 , 157–176. [ Google Scholar ]
- Sánchez, Ó.J.; Ospina, D.A.; Montoya, S. Compost supplementation with nutrients and microorganisms in composting process. Waste Manag. 2017 , 69 , 136–153. [ Google Scholar ] [ CrossRef ]
- Azim, K.; Soudi, B.; Boukhari, S.; Perissol, C.; Roussos, S.; Alami, I.T. Composting parameters and compost quality: A literature review. Org. Agric. 2018 , 8 , 141–158. [ Google Scholar ] [ CrossRef ]
- Li, R.; Wang, J.J.; Zhang, Z.; Shen, F.; Zhang, G.; Qin, R.; Li, X.; Xiao, R. Nutrient transformations during composting of pig manure with bentonite. Bioresour. Technol. 2012 , 121 , 362–368. [ Google Scholar ] [ CrossRef ] [ PubMed ]
- Akdeniz, N. A systematic review of biochar use in animal waste composting. Waste Manag. 2019 , 88 , 291–300. [ Google Scholar ] [ CrossRef ] [ PubMed ]
- Shan, G.; Xu, J.; Jiang, Z.; Li, M.; Li, Q. The transformation of different dissolved organic matter sub fractions and distribution of heavy metals during food waste and sugarcane leaves co-composting. Waste Manag. 2019 , 87 , 636–644. [ Google Scholar ] [ PubMed ]
- Zhang, L.; Sun, X. Evaluation of maifanite and silage as amendments for green waste composting. Waste Manag. 2018 , 77 , 435–446. [ Google Scholar ] [ CrossRef ] [ PubMed ]
- Soobhany, N.; Mohee, R.; Garg, V.K. Recovery of nutrient from Municipal Solid Waste by composting and vermicomposting using earthworm Eudrilus eugeniae. J. Environ. Chem. Eng. 2015 , 3 , 2931–2942. [ Google Scholar ] [ CrossRef ]
- Tiquia, S.M.; Richard, T.L.; Honeyman, M.S. Carbon, nutrient, and mass loss during composting. Nutr. Cycl. Agroecosyst. 2002 , 62 , 15–24. [ Google Scholar ] [ CrossRef ]
- Sánchez, A. A kinetic analysis of solid waste composting at optimal conditions. Waste Manag. 2006 , 27 , 854–855. [ Google Scholar ] [ CrossRef ] [ Green Version ]
- Ruggieri, L.; Gea, T.; Artola, A.; Sánchez, A. Air filled porosity measurements by air pycnometry in the composting process: A review and a correlation analysis. Bioresour. Technol. 2009 , 100 , 2655–2666. [ Google Scholar ] [ CrossRef ]
- Niwagaba, C.; Nalubega, M.; Vinnerås, B.; Sundberg, C.; Jonsson, H. Bench-scale composting of source-separated human faeces for sanitation. Waste Manag. 2009 , 29 , 585–589. [ Google Scholar ] [ CrossRef ]
- Hsu, J.-H.; Lo, S.-L. Chemical and spectroscopic analysis of organic matter transformations during composting of pig manure. Environ. Pollut. 1999 , 104 , 189–196. [ Google Scholar ] [ CrossRef ]
- Liu, X.; Rashti, M.R.; Dougall, A.; Esfandbod, M.; Van Zwieten, L.; Chen, C. Subsoil application of compost improved sugarcane yield through enhanced supply and cycling of soil labile organic carbon and nitrogen in an acidic soil at tropical Australia. Soil Tillage Res. 2018 , 180 , 73–81. [ Google Scholar ] [ CrossRef ]
- Liu, L.; Wang, S.; Guo, X.; Wang, H. Comparison of the effects of different maturity composts on soil nutrient, plant growth and heavy metal mobility in the contaminated soil. J. Environ. Manag. 2019 , 250 , 109525. [ Google Scholar ] [ CrossRef ]
- Huang, G.; Wu, Q.; Wong, J.W.C.; Nagar, B. Transformation of organic matter during co-composting of pig manure with sawdust. Bioresour. Technol. 2006 , 97 , 1834–1842. [ Google Scholar ] [ CrossRef ] [ PubMed ]
- Daniel, F.; Bruno, G. Synergisms between Compost and Biochar for Sustainable Soil Amelioration, Management of Organic Waste ; Sunil, K., Ed.; InTech Europe: Rijeka, Croatia, 2012; Available online: http://www.intechopen.com (accessed on 15 March 2020).
- Bouajila, K.; Sanaa, M. Effects of organic amendments on soil physico-chemical and biological properties. J. Mater. Environ. Sci. 2011 , 2 , 485–490. [ Google Scholar ]
- Flavel, T.; Murphy, D.V.; Lalor, B.; Fillery, I. Gross N mineralization rates after application of composted grape marc to soil. Soil Biol. Biochem. 2005 , 37 , 1397–1400. [ Google Scholar ] [ CrossRef ]
- Komilis, D.; Kontou, I.; Ntougias, S. A modified static respiration assay and its relationship with an enzymatic test to assess compost stability and maturity. Bioresour. Technol. 2011 , 102 , 5863–5872. [ Google Scholar ] [ CrossRef ]
- Sarsaiya, S.; Jain, A.; Awasthi, S.K.; Duan, Y.; Awasthi, M.K.; Shi, J. Microbial dynamics for lignocellulosic waste bioconversion and its importance with modern circular economy, challenges and future perspectives. Bioresour. Technol. 2019 , 291 , 121905. [ Google Scholar ] [ CrossRef ]
- Sarsaiya, S.; Jia, Q.; Fan, X.; Jain, A.; Shu, F.; Lu, Y.; Shi, J.; Chen, J. First report of leaf black circular spots on Dendrobiumnobile caused by Trichoderma longibrachiatum in Guizhou Province, China. Plant Dis. 2019 , 103 , 3275. [ Google Scholar ]
- Cerda, A.; Artola, A.; Font, X.; Barrena, R.; Gea, T.; Sánchez, A. Composting of food wastes: Status and challenges. Bioresour. Technol. 2018 , 248 , 57–67. [ Google Scholar ] [ CrossRef ] [ Green Version ]
- Bernal, M.; Alburquerque, J.; Moral, R. Composting of animal manures and chemical criteria for compost maturity assessment. A review. Bioresour. Technol. 2009 , 100 , 5444–5453. [ Google Scholar ] [ CrossRef ]
- Komilis, D.; Tziouvaras, I.S. A statistical analysis to assess the maturity and stability of six composts. Waste Manag. 2009 , 29 , 1504–1513. [ Google Scholar ] [ CrossRef ] [ PubMed ]
- Raj, D.; Antil, R. Evaluation of maturity and stability parameters of composts prepared from agro-industrial wastes. Bioresour. Technol. 2011 , 102 , 2868–2873. [ Google Scholar ] [ CrossRef ] [ PubMed ]
- Adani, F.; Confalonieri, R.; Tambone, F. Dynamic Respiration Index as a Descriptor of the Biological Stability of Organic Wastes. J. Environ. Qual. 2004 , 33 , 1866–1876. [ Google Scholar ] [ CrossRef ] [ PubMed ]
- Guo, R.; Li, G.; Jiang, T.; Schuchardt, F.; Chen, T.; Zhao, Y.; Shen, Y. Effect of aeration rate, C/N ratio and moisture content on the stability and maturity of compost. Bioresour. Technol. 2012 , 112 , 171–178. [ Google Scholar ] [ CrossRef ] [ PubMed ]
- Adani, F.; Gigliotti, G.; Valentini, F.; Laraia, R. Respiration Index Determination: A Comparative Study of Different Methods. Compos. Sci. Util. 2003 , 11 , 144–151. [ Google Scholar ] [ CrossRef ]
- Barrena, R.; D’Imporzano, G.; Ponsá, S.; Gea, T.; Artola, A.; Vázquez, F.; Sánchez, A.; Adani, F. In search of a reliable technique for the determination of the biological stability of the organic matter in the mechanical–biological treated waste. J. Hazard. Mater. 2009 , 162 , 1065–1072. [ Google Scholar ] [ CrossRef ] [ Green Version ]
- Cai, A.; Xu, M.; Wang, B.; Zhang, W.; Liang, G.; Hou, E.; Luo, Y. Manure acts as a better fertilizer for increasing crop yields than synthetic fertilizer does by improving soil fertility. Soil Tillage Res. 2019 , 189 , 168–175. [ Google Scholar ] [ CrossRef ]
- Ye, G.; Lin, Y.; Liu, D.; Chen, Z.; Luo, J.; Bolan, N.; Fa, J.; Ding, W. Long-term application of manure over plant residues mitigates acidification, builds soil organic carbon and shifts prokaryotic diversity in acidic Ultisols. Appl. Soil Ecol. 2019 , 133 , 24–33. [ Google Scholar ] [ CrossRef ]
- Duong, T.T.T.; Penfold, C.; Marschner, P. Differential effects of composts on properties of soils with different textures. Biol. Fertil. Soils 2012 , 48 , 699–707. [ Google Scholar ] [ CrossRef ]
- Huang, P.M.; Li, Y.; Sumner, M.E. Land Application of Wastes. In Handbook of Soil Sciences ; CRC Press: Boca Raton, FL, USA, 2011; pp. 620–645. [ Google Scholar ]
- Tittarelli, F.; Petruzzelli, G.; Pezzarossa, B.; Civilini, M.; Benedetti, A.; Sequi, P. Chapter 7 Quality and agronomic use of compost. In Solid Waste: Assessment, Monitoring and Remediation ; Elsevier: Amsterdam, The Netherlands, 2007; pp. 119–157. [ Google Scholar ]
- Rady, M.M.; Semida, W.M.; Hemida, K.A.; Abdelhamid, M.T. The effect of compost on growth and yield of Phaseolus vulgaris plants grown under saline soil. Int. J. Recycl. Org. Waste Agric. 2016 , 5 , 311–321. [ Google Scholar ] [ CrossRef ] [ Green Version ]
- Adugna, G. A review on impact of compost on soil properties, water use and crop productivity. Acad. Res. J. Agric. Sci. Res. 2016 , 4 , 93–104. [ Google Scholar ]
- Amlinger, F.; Peyr, S.; Geszit, J.; Dreher, P.; Weinfurtner, K.; Nortcliff, S. Beneficial effects of compost application on fertility and productivity of soils: Literature study. Report produced for the Federal Ministry of Agriculture and Forestry. Environ. Water Manag. 2007 . Available online: http://www.umweltnet.at/article/articlereview/51825/1/6954/ (accessed on 22 November 2020).
- Richard, T.L. Compost. In Encyclopedia of Soils in the Environment ; Elsevier: Oxford, UK, 2005; pp. 294–301. [ Google Scholar ]
- De Bertoldi, M. The Science of Composting ; Springer: Berlin/Heidelberg, Germany, 2013. [ Google Scholar ]
- Chandna, P.; Nain, L.; Singh, S.; Kuhad, R.C. Assessment of bacterial diversity during composting of agricultural byproducts. BMC Microbiol. 2013 , 13 , 1–14. [ Google Scholar ] [ CrossRef ] [ Green Version ]
- Confesor, R.; Hamlett, J.; Shannon, R.; Graves, R. Potential Pollutants from Farm, Food and Yard Waste Composts at Differing Ages: Leaching Potential of Nutrients under Column Experiments. Part II. Compos. Sci. Util. 2009 , 17 , 6–17. [ Google Scholar ] [ CrossRef ]
- Neher, D.A.; Weicht, T.R.; Bates, S.T.; Leff, J.W.; Fierer, N. Changes in Bacterial and Fungal Communities across Compost Recipes, Preparation Methods, and Composting Times. PLoS ONE 2013 , 8 , e79512. [ Google Scholar ] [ CrossRef ] [ Green Version ]
- Cesaro, A.; Belgiorno, V.; Guida, M. Compost from organic solid waste: Quality assessment and European regulations for its sustainable use. Resour. Conserv. Recycl. 2015 , 94 , 72–79. [ Google Scholar ] [ CrossRef ]
- Muscolo, A.; Papalia, T.; Settineri, G.; Mallamaci, C.; Jeske-Kaczanowska, A. Are raw materials or composting conditions and time that most influence the maturity and/or quality of composts? Comparison of obtained composts on soil properties. J. Clean. Prod. 2018 , 195 , 93–101. [ Google Scholar ] [ CrossRef ]
- Bertoncini, E.; D’Orazio, V.; Senesi, N.; Mattiazzo, M. Effects of sewage sludge amendment on the properties of two Brazilian oxisols and their humic acids. Bioresour. Technol. 2008 , 99 , 4972–4979. [ Google Scholar ] [ CrossRef ]
- Jorge-Mardomingo, I.; Jiménez-Hernández, M.E.; Moreno, L.; De La Losa, A.; De La Cruz, M.T.; Casermeiro, M. Ángel Application of high doses of organic amendments in a Mediterranean agricultural soil: An approach for assessing the risk of groundwater contamination. Catena 2015 , 131 , 74–83. [ Google Scholar ] [ CrossRef ]
- Perego, A.; Basile, A.; Bonfante, A.; De Mascellis, R.; Terribile, F.; Brenna, S.; Acutis, M. Nitrate leaching under maize cropping systems in Po Valley (Italy). Agric. Ecosyst. Environ. 2012 , 147 , 57–65. [ Google Scholar ] [ CrossRef ]
- Ko, H.J.; Kim, K.Y.; Kim, H.T.; Kim, C.N.; Umeda, M. Evaluation of maturity parameters and heavy metal contents in composts made from animal manure. Waste Manag. 2008 , 28 , 813–820. [ Google Scholar ] [ CrossRef ] [ PubMed ]
- Alvarenga, P.; Mourinha, C.; Farto, M.; Santos, T.; Palma, P.; Sengo, J.; Morais, M.-C.; Cunha-Queda, C. Sewage sludge, compost and other representative organic wastes as agricultural soil amendments: Benefits versus limiting factors. Waste Manag. 2015 , 40 , 44–52. [ Google Scholar ] [ CrossRef ]
- Ling, N.; Xue, C.; Huang, Q.; Yang, X.; Xu, Y.; Shen, Q. Development of a mode of application of bioorganic fertilizer for improving the biocontrol efficacy to Fusarium wilt. BioControl 2010 , 55 , 673–683. [ Google Scholar ] [ CrossRef ]
- Said-Pullicino, D.; Kaiser, K.; Guggenberger, G.; Gigliotti, G. Changes in the chemical composition of water-extractable organic matter during composting: Distribution between stable and labile organic matter pools. Chemosphere 2007 , 66 , 2166–2176. [ Google Scholar ] [ CrossRef ]
- Giorgi, G. Managing soil nutrients with compost in organic farms of East Georgia. EGU Gen. Assem. 2013 , 15 , 11640. [ Google Scholar ]
- Garcia, C.; Hernandez, T.; Coll, M.D.; Ondoño, S. Organic amendments for soil restoration in arid and semiarid areas: A review. ATM Environ. Sci. 2017 , 4 , 640–676. [ Google Scholar ]
- Bünemann, E.K.; Bongiorno, G.; Bai, Z.; Creamer, R.E.; De Deyn, G.; De Goede, R.; Fleskens, L.; Geissen, V.; Kuyper, T.W.; Mäder, P.; et al. Soil quality—A critical review. Soil Biol. Biochem. 2018 , 120 , 105–125. [ Google Scholar ] [ CrossRef ]
- Li, J.; Cooper, J.M.; Lin, Z.; Li, Y.; Yang, X.; Zhao, B. Soil microbial community structure and function are significantly affected by long-term organic and mineral fertilization regimes in the North China Plain. Appl. Soil Ecol. 2015 , 96 , 75–87. [ Google Scholar ] [ CrossRef ]
- Nannipieri, P.; Trasar-Cepeda, C.; Dick, R.P. Soil enzyme activity: A brief history and biochemistry as a basis for appropriate interpretations and meta-analysis. Biol. Fertil. Soils 2018 , 54 , 11–19. [ Google Scholar ] [ CrossRef ]
- Medina, A.; Vassilev, N.; Alguacil, M.; Roldán, A.; Azcón, R. Increased plant growth, nutrient uptake, and soil enzymatic activities in a desertified Mediterranean soil amended with treated residues and inoculated with native mycorrhizal fungi and a plant growth-promoting yeast. Soil Sci. 2004 , 169 , 260–270. [ Google Scholar ]
- Lupwayi, N.Z.; Zhang, Y.; Hao, X.; Thomas, B.W.; Eastman, A.H.; Schwinghamer, T.D. Linking soil microbial biomass and enzyme activities to long-term manure applications and their nonlinear legacy. Pedobiology 2019 , 74 , 34–42. [ Google Scholar ] [ CrossRef ]
- Bastida, F.; Kandeler, E.; Hernandez, T.; Garcia, C. Long-term Effect of Municipal Solid Waste Amendment on Microbial Abundance and Humus-associated Enzyme Activities under Semiarid Conditions. Microb. Ecol. 2007 , 55 , 651–661. [ Google Scholar ] [ CrossRef ]
- Leon, M.C.C.; Stone, A.; Dick, R.P. Organic soil amendments: Impacts on snap bean common root rot (Aphanomyes euteiches) and soil quality. Appl. Soil Ecol. 2006 , 31 , 199–210. [ Google Scholar ] [ CrossRef ]
- Dukare, A.S.; Prasanna, R.; Dubey, S.C.; Nain, L.; Chaudhary, V.; Singh, R.; Saxena, A.K. Evaluating novel microbe amended composts as biocontrol agents in tomato. Crop. Prot. 2011 , 30 , 436–442. [ Google Scholar ] [ CrossRef ]
- Zhen, Z.; Liu, H.; Wang, N.; Guo, L.; Meng, J.; Ding, N.; Wu, G.; Jiang, G.M. Effects of Manure Compost Application on Soil Microbial Community Diversity and Soil Microenvironments in a Temperate Cropland in China. PLoS ONE 2014 , 9 , e108555. [ Google Scholar ] [ CrossRef ]
- Oehl, F.; Sieverding, E.; Dubois, D.; Ineichen, K.; Boller, T.; Wiemken, A. Impact of long-term conventional and organic farming on the diversity of arbuscular mycorrhizal fungi. Oecologia 2004 , 138 , 574–583. [ Google Scholar ] [ CrossRef ]
- Gosling, P.; Hodge, A.; Goodlass, G.; Bending, G. Arbuscular mycorrhizal fungi and organic farming. Agric. Ecosyst. Environ. 2006 , 113 , 17–35. [ Google Scholar ] [ CrossRef ]
- Clark, R.B.; Zeto, S.K. Mineral acquisition by arbuscular mycorrhizal plants. J. Plant Nutr. 2000 , 23 , 867–902. [ Google Scholar ] [ CrossRef ]
- Cornejo, P.; Meier, S.; Borie, G.; Rillig, M.C.; Borie, F. Glomalin-related soil protein in a Mediterranean ecosystem affected by a copper smelter and its contribution to Cu and Zn sequestration. Sci. Total Environ. 2008 , 406 , 154–160. [ Google Scholar ] [ CrossRef ]
- Ginting, D.; Kessavalou, A.; Eghball, B.; Doran, J.W. Greenhouse gas emissions and soil indicators four years after manure and compost applications. J. Environ. Qual. 2003 , 32 , 23–32. [ Google Scholar ]
- Paulin, B.; Peter, O.M. Compost Production and Use in Horticulture. West. Aust. Agric. Auth. 2008 . Available online: www.agric.wa.gov.au (accessed on 10 March 2020).
- Osman, K.T. Physical Properties of Soil. In Soils ; Springer: Berlin/Heidelberg, Germany, 2012; pp. 49–65. [ Google Scholar ]
- Novotná, J.; Badalíková, B. The Soil Structure Changes under Varying Compost Dosage. Agriculture 2018 , 64 , 143–148. [ Google Scholar ] [ CrossRef ] [ Green Version ]
- Scotti, R.; Bonanomi, G.; Scelza, R.; Zoina, A.; Rao, M. Organic amendments as sustainable tool to recovery fertility in intensive agricultural systems. J. Soil Sci. Plant Nutr. 2015 , 15 , 333–352. [ Google Scholar ] [ CrossRef ] [ Green Version ]
- Duong, T.T. Compost effects on soil properties and plant growth. EGU Gen. Assem. 2014 , 2013–2797. [ Google Scholar ]
- Annabi, M.; Houot, S.; Francou, C.; Poitrenaud, M.; Le Bissonnais, Y. Soil Aggregate Stability Improvement with Urban Composts of Different Maturities. Soil Sci. Soc. Am. J. 2007 , 71 , 413–423. [ Google Scholar ] [ CrossRef ]
- Ozlu, E.; Kumar, S. Response of Soil Organic Carbon, pH, Electrical Conductivity, and Water Stable Aggregates to Long-Term Annual Manure and Inorganic Fertilizer. Soil Sci. Soc. Am. J. 2018 , 82 , 1243–1251. [ Google Scholar ] [ CrossRef ]
- Kroulík, M.; Brant, V.; Masek, J.; Kovaříček, P. Influence of soil tillage treatment and compost application on soil properties and water infiltration. In Trends in Agricultural Engineering, Proceedings of the 4th International Conference ; Czech University of Life Sciences: Prague, Czech, 2010; pp. 343–349. ISBN 978-80-213-2088-8. [ Google Scholar ]
- Martínez-Blanco, J.; Lazcano, C.; Christensen, T.H.; Muñoz, P.; Rieradevall, J.; Møller, J.; Antón, A.; Boldrin, A. Compost benefits for agriculture evaluated by life cycle assessment. A review. Agron. Sustain. Dev. 2013 , 33 , 721–732. [ Google Scholar ] [ CrossRef ] [ Green Version ]
- Zou, C.; Li, Y.; Huang, W.; Zhao, G.K.; Pu, G.; Su, J.; Coyne, M.S.; Chen, Y.; Wang, L.; Hu, X.; et al. Rotation and manure amendment increase soil macro-aggregates and associated carbon and nitrogen stocks in flue-cured tobacco production. Geoderma 2018 , 325 , 49–58. [ Google Scholar ] [ CrossRef ]
- Babalola, O.; Adesodun, J.; Olasantan, F.; Adekunle, A. Responses of Some Soil Biological, Chemical and Physical Properties to Short-term Compost Amendment. Int. J. Soil Sci. 2011 , 7 , 28–38. [ Google Scholar ] [ CrossRef ] [ Green Version ]
- Annabi, M.; Le Bissonnais, Y.; Le Villio-Poitrenaud, M.; Houot, S. Improvement of soil aggregate stability by repeated applications of organic amendments to a cultivated silty loam soil. Agric. Ecosyst. Environ. 2011 , 144 , 382–389. [ Google Scholar ] [ CrossRef ]
- Martens, D.A.; Frankenberger, W.T. Modification of Infiltration Rates in an Organic-Amended Irrigated. Agron. J. 1907 , 84 , 707–717. [ Google Scholar ] [ CrossRef ]
- Crogger, C.G. Potential compost benefits for restoration of soils disturbed by urban development. Compost. Sci. Util. 2005 , 13 , 243–251. [ Google Scholar ]
- Kranz, C.N.; McLaughlin, R.A.; Johnson, A.; Miller, G.; Heitman, J.L. The effects of compost incorporation on soil physical properties in urban soils—A concise review. J. Environ. Manag. 2020 , 261 , 110209. [ Google Scholar ] [ CrossRef ]
- Carrizo, M.E.; Alesso, C.A.; Cosentino, D.; Imhoff, S. Aggregation agents and structural stability in soils with different texture and organic carbon contents. Sci. Agric. 2015 , 72 , 75–82. [ Google Scholar ] [ CrossRef ] [ Green Version ]
- Brown, S.; Cotton, M. Changes in Soil Properties and Carbon Content Following Compost Application: Results of On-farm Sampling. Compos. Sci. Util. 2011 , 19 , 87–96. [ Google Scholar ] [ CrossRef ]
- Curtis, M.J.; Claassen, V.P. Regenerating Topsoil Functionality in Four Drastically Disturbed Soil Types by Compost Incorporation. Restor. Ecol. 2009 , 17 , 24–32. [ Google Scholar ] [ CrossRef ]
- Somerville, P.D.; May, P.B.; Livesley, S.J. Effects of deep tillage and municipal green waste compost amendments on soil properties and tree growth in compacted urban soils. J. Environ. Manag. 2018 , 227 , 365–374. [ Google Scholar ] [ CrossRef ]
- Cogger, C.; Hummel, R.; Hart, J.; Bary, A. Soil and Redosier Dogwood Response to Incorporated and Surface-applied Compost. HortScience 2008 , 43 , 2143–2150. [ Google Scholar ] [ CrossRef ] [ Green Version ]
- Leroy, B.L.M.; Herath, H.M.S.K.; Sleutel, S.; De Neve, S.; Gabriëls, D.; Reheul, D.; Moens, M. The quality of exogenous organic matter: Short-term effects on soil physical properties and soil organic matter fractions. Soil Use Manag. 2008 , 24 , 139–147. [ Google Scholar ] [ CrossRef ]
- Zebarth, B.J.; Neilsen, G.H.; Hogue, E.; Neilsen, D. Influence of organic waste amendments on selected soil physical and chemical properties. Can. J. Soil Sci. 1999 , 79 , 501–504. [ Google Scholar ] [ CrossRef ]
- Badalíková, B.; Bartlová, J. Influence of compost incorporated on the soil compaction. Sci. Suppl. 2011 , 12 , 311–314, (In Úroda). [ Google Scholar ]
- Skuras, D.; Psaltopoulos, D. A broad overview of the main problems derived from climate change that will affect agricultural production in the Mediterranean area. In Building Resilience for Adaptation to Climate Change in the Agriculture Sector: Proceedings of a Joint FAO/OECD Workshop ; 2012; pp. 217–260. Available online: http://www.fao.org/3/i3084e/i3084e.pdf (accessed on 22 November 2020).
- Weindorf, D.C.; Zartman, R.E.; Allen, B. Effect of Compost on Soil Properties in Dallas, Texas. Compos. Sci. Util. 2006 , 14 , 59–67. [ Google Scholar ] [ CrossRef ]
- Logsdon, S.D.; Sauer, P.A.; Shipitalo, M. Compost Improves Urban Soil and Water Quality. J. Water Resour. Prot. 2017 , 9 , 345–357. [ Google Scholar ] [ CrossRef ] [ Green Version ]
- Vengadaramana, A.; Justin, P.T.J. Effect of organic fertilizers on the water holding capacity of soil in different terrains of Jaffna peninsula in Sri Lanka. J. Nat. Prod. Plant Resour. 2012 , 2 , 500–503. [ Google Scholar ]
- Schmid, C.; Murphy, J. Effect of tillage and compost amendment on turfgrass establishment on a compacted sandy loam. J. Soil Water Conserv. 2016 , 72 , 55–64. [ Google Scholar ] [ CrossRef ]
- Aggelides, S.; Londra, P. Effects of compost produced from town wastes and sewage sludge on the physical properties of a loamy and a clay soil. Bioresour. Technol. 2000 , 71 , 253–259. [ Google Scholar ] [ CrossRef ]
- Edwards, S.; Hailu, A. How to make compost and use. In Climate Change and Food Systems Resilience in Sub-Sarahan Africa ; Ching, L.L., Edwards, S., Nadia, H.S., Eds.; FAO: Rome, Italy, 2011; pp. 379–436. [ Google Scholar ]
- Johns, C. The Chemical Fertility of Soils: Soil Nutrients and Plant Nutrition ; Future Direction International Pty Ltd.: Nedlands, Australia, 2015. [ Google Scholar ]
- Choi, K. Optimal operating parameters in the composting of swine manure with wastepaper. J. Environ. Sci. Heal. Part B 1999 , 34 , 975–987. [ Google Scholar ] [ CrossRef ]
- Masmoudi, S.; Magdich, S.; Rigane, H.; Medhioub, K.; Rebai, A.; Ammar, E. Effects of Compost and Manure Application Rate on the Soil Physico-Chemical Layers Properties and Plant Productivity. Waste Biomass Valoriz. 2018 , 11 , 1883–1894. [ Google Scholar ] [ CrossRef ]
- Schlegel, A.J. Effect of Composted Manure on Soil Chemical Properties and Nitrogen Use by Grain Sorghum. J. Prod. Agric. 1992 , 5 , 153–157. [ Google Scholar ] [ CrossRef ]
- Eghball, B. Nitrogen Mineralization from Field-Applied Beef Cattle Feedlot Manure or Compost. Soil Sci. Soc. Am. J. 2000 , 64 , 2024–2030. [ Google Scholar ] [ CrossRef ]
- Eghball, B.; Power, J.F. Phosphorus- and Nitrogen-Based Manure and Compost Applications Corn Production and Soil Phosphorus. Soil Sci. Soc. Am. J. 1999 , 63 , 895–901. [ Google Scholar ] [ CrossRef ] [ Green Version ]
- Amlinger, F.; Götz, B.; Dreher, P.; Geszti, J.; Weissteiner, C. Nitrogen in biowaste and yard waste compost: Dynamics of mobilisation and availability—A review. Eur. J. Soil Biol. 2003 , 39 , 107–116. [ Google Scholar ] [ CrossRef ]
- Diacono, M.; Montemurro, F. Long-term effects of organic amendments on soil fertility. A review. Agron. Sustain. Dev. 2010 , 30 , 401–422. [ Google Scholar ] [ CrossRef ] [ Green Version ]
- Bhogal, A.; Chambers, B.J.; Whitmore, A.P.; Powlson, D.S. The Effect of Reduced Tillage Practices and Organic Matter Additions on the Carbon Content of Arable Soils ; Scientific Report SP0561; Department of Environment, Food and Rural Affairs: London, UK, 2007; p. 47.
- Bendfeld, J.; Tigges, M.; Splett, M.; Voss, J. GHG Savings from Biological Treatment and Application of Compost ORBIT 2008. In Proceedings of the 6th International Conference: ORBIT 2008-Moving Organic Waste Recycling towards Resource Management and for Biobased Economy, Wageningen, The Netherlands, 13–15 October 2008; pp. 626–631. [ Google Scholar ]
- Smith, A.; Brown, K.; Ogilvie, S.; Rushton, K.; Bates, J. Waste Management Options and Climate Change ; European Commission: Abingdon, UK, 2001; p. 224. [ Google Scholar ]
- Gallardo-Lara, F.; Nogals, R. Effect of the application of town refuse compost on the soil-plant system: A review. Biol. Wastes 1987 , 19 , 35–62. [ Google Scholar ] [ CrossRef ]
- Atiyeh, R.M.; Edwards, C.A.; Subler, S.; Metzgerc, J.D. Pig manurevermicompost as a component of a horticultural bedding plant medium: Effects on physicochemical properties and plant growth. Bioresour. Technol. 2001 , 78 , 11–20. [ Google Scholar ]
- Sarwar, G.; Hussain, N.; Mujeeb, F.; Schmeisky, H.; Hassan, G. Biocompost Application for the Improvement of Soil Characteristics and Dry Matter Yield of Lolium perenne (Grass). Asian J. Plant Sci. 2003 , 2 , 237–241. [ Google Scholar ] [ CrossRef ]
- Niklasch, H.; Joergensen, R.G. Decomposition of peat, biogenic municipal waste compost, and shrub/grass compost added in different rates to a silt loam. J. Plant Nutr. Soil Sci. 2001 , 164 , 365–369. [ Google Scholar ] [ CrossRef ]
- Gonzalez, M.; Gomez, E.; Comese, R.; Quesada, M.; Conti, M. Influence of Organic Amendments on Soil Quality Potential Indicators in an Urban Horticultural Sys-tem. Bioresour. Technol. 2010 , 101 , 8897–8901. [ Google Scholar ]
- Shiralipour, A.; McConnell, D.B.; Smith, W.H. Physical and chemical properties of soils as affected by municipal solid waste compost application. Biomass Bioenerg. 1992 , 3 , 261–266. [ Google Scholar ]
- Walker, D.J.; Clemente, R.; Roig, A.; Bernal, M. The effects of soil amendments on heavy metal bioavailability in two contaminated Mediterranean soils. Environ. Pollut. 2003 , 122 , 303–312. [ Google Scholar ] [ CrossRef ]
- Smiciklas, K.D.; Walker, P.M.; Kelley, P.M. Utilization of Compost (Food, Paper, Landscape and Manure) in Row Crop Production ; Illinois State University: Normal, IL, USA, 2002. [ Google Scholar ]
- Yaduvanshi, N.P.S. Effect of five years of rice-wheat cropping and NPK fertilizer use with and without organic and green manures on soil properties and crop yields in a reclaimed sodic soil. J. Indian Soc. Soil Sci. 2001 , 49 , 714–719. [ Google Scholar ]
- Taylor, M.D.; Kreis, R.; Rejtö, L. Establishing Growing Substrate pH with Compost and Limestone and the Impact on pH Buffering Capacity. HortScience 2016 , 51 , 1153–1158. [ Google Scholar ] [ CrossRef ] [ Green Version ]
- Lima, J.; De Queiroz, J.; Freitas, H. Effect of selected and non-selected urban waste compost on the initial growth of corn. Resour. Conserv. Recycl. 2004 , 42 , 309–315. [ Google Scholar ] [ CrossRef ]
- Gharib, F.A.; Moussa, L.A.; Massoud, O.N. Effect of compost and bio-fertilizers on growth, yield and essential oil of sweet marjoram (Majorana hortensis) plant. Int. J. Agric. Biol. 2008 , 10 , 381–387. [ Google Scholar ]
- Asses, N.; Farhat, W.; Hamdi, M.; Bouallagui, H. Large scale composting of poultry slaughterhouse processing waste: Microbial removal and agricultural biofertilizer application. Process. Saf. Environ. Prot. 2019 , 124 , 128–136. [ Google Scholar ] [ CrossRef ]
- Majbar, Z.; Lahlou, K.; Ben Abbou, M.; Ammar, E.; Triki, A.; Abid, W.; Nawdali, M.; Bouka, H.; Taleb, M.; El Haji, M.; et al. Co-composting of Olive Mill Waste and Wine-Processing Waste: An Application of Compost as Soil Amendment. J. Chem. 2018 , 2018 , 1–9. [ Google Scholar ] [ CrossRef ]
- Moral, R.; Paredes, C.; Bustamante, M.; Marhuenda-Egea, F.; Bernal, M. Utilisation of manure composts by high-value crops: Safety and environmental challenges. Bioresour. Technol. 2009 , 100 , 5454–5460. [ Google Scholar ] [ CrossRef ]
- Borrero, C.; Trillas, M.I.; Ordovás, J.; Tello, J.C.; Avilés, M. Predictive Factors for the Suppression of Fusarium Wilt of Tomato in Plant Growth Media. Phytopathology 2004 , 94 , 1094–1101. [ Google Scholar ] [ CrossRef ] [ Green Version ]
- Aviles, M.; Borrero, C.; Trillas, M. Review on compost as an inducer of disease suppression in plants grown in soilless culture. Dyn. Soil Dyn. Plant 2011 , 5 , 1–11. [ Google Scholar ]
- Singh, R.; Singh, R.; Soni, S.K.; Singh, S.P.; Chauhan, U.; Kalra, A. Vermicompost from biodegraded distillation waste improves soil properties and essential oil yield of Pogostemon cablin (patchouli) Benth. Appl. Soil Ecol. 2013 , 70 , 48–56. [ Google Scholar ] [ CrossRef ]
- De Corato, U. Agricultural waste recycling in horticultural intensive farming systems by on-farm composting and compost-based tea application improves soil quality and plant health: A review under the perspective of a circular economy. Sci. Total Environ. 2020 , 738 , 139840. [ Google Scholar ] [ CrossRef ] [ PubMed ]
- Ballardo, C.; Vargas-García, M.D.C.; Sánchez, A.; Barrena, R.; Artola, A. Adding value to home compost: Biopesticide properties through Bacillus thuringiensis inoculation. Waste Manag. 2020 , 106 , 32–43. [ Google Scholar ] [ CrossRef ] [ PubMed ]
- Loffredo, E.; Berloco, M.; Senesi, N. The role of humic fractions from soil and compost in controlling the growth in vitro of phytopathogenic and antagonistic soil-borne fungi. Ecotoxicol. Environ. Saf. 2008 , 69 , 350–357. [ Google Scholar ] [ CrossRef ] [ PubMed ]
- Dionne, A.; Tweddell, R.J.; Antoun, H.; Avis, T.J. Effect of non-aerated compost teas on damping-off pathogens of tomato. Can. J. Plant Pathol. 2012 , 34 , 51–57. [ Google Scholar ] [ CrossRef ]
- Pascual, J.A.; Garcia, C.; Hernandez, T.; Lerma, S.; Lynch, J.M. Effectiveness of municipal waste compost and its humic fraction in suppressing Pythium ultimum. Microb. Ecol. 2002 , 44 , 59–68. [ Google Scholar ] [ CrossRef ] [ PubMed ]
- Alfano, G.; Lustrato, G.; Lima, G.; Vitullo, D.; Ranalli, G. Characterization of composted olive mill wastes to predict potential plant disease suppressiveness. Biol. Control 2011 , 58 , 199–207. [ Google Scholar ] [ CrossRef ]
- Litterick, A.M.; Harrier, L.; Wallace, P.; Watson, C.A.; Wood, M. The role of uncomposted materials, composts, manures and compost extracts in reducing pest and disease incidence and severity in sustainable temperate agricultural and horticultural crop production—A review. Crit. Rev. Plant Sci. 2004 , 23 , 453–479. [ Google Scholar ]
- Tong, J.; Sun, X.; Li, S.; Qu, B.; Wan, L. Reutilization of Green Waste as Compost for Soil Improvement in the Afforested Land of the Beijing Plain. Sustainability 2018 , 10 , 2376. [ Google Scholar ] [ CrossRef ] [ Green Version ]
- Sax, M.S.; Bassuk, N.; Van Es, H.; Rakow, D. Long-term remediation of compacted urban soils by physical fracturing and incorporation of compost. Urban For. Urban Green. 2017 , 24 , 149–156. [ Google Scholar ] [ CrossRef ]
- Jindo, K.; Chocano, C.; De Aguilar, J.M.; González, D.; Hernandez, T.; García, C. Impact of Compost Application during 5 Years on Crop Production, Soil Microbial Activity, Carbon Fraction, and Humification Process. Commun. Soil Sci. Plant Anal. 2016 , 47 , 1907–1919. [ Google Scholar ] [ CrossRef ]
- Cannavo, P.; Vidal-Beaudet, L.; Grosbellet, C. Prediction of long-term sustainability of constructed urban soil: Impact of high amounts of organic matter on soil physical properties and water transfer. Soil Use Manag. 2014 , 30 , 272–284. [ Google Scholar ] [ CrossRef ]
- Fuchs, J.; Fliessbach, A.; Mader, P.; Weibel, F.; Tamm, L.; Mayer, J.; Schleiss, K. Effects of Compost on Soil Fertility Parameters In Short-, Mid- And Long-Term Field Experiments. Acta Hortic. 2014 , 39–46. [ Google Scholar ] [ CrossRef ]
- Habteselassie, M.Y.; Miller, B.E.; Thacker, S.G.; Stark, J.M.; Norton, J.M. Soil Nitrogen and Nutrient Dynamics after Repeated Application of Treated Dairy-Waste. Soil Sci. Soc. Am. J. 2006 , 70 , 1328–1337. [ Google Scholar ] [ CrossRef ]
- Whalen, J.K.; Benslim, H.; Jiao, Y.; Sey, B.K. Soil organic carbon and nitrogen pools as affected by compost applicationsto a sandy-loam soil in Québec. Can. J. Soil Sci. 2008 , 88 , 443–450. [ Google Scholar ] [ CrossRef ] [ Green Version ]
- Curtis, M.J.; Claassen, V.P. Compost Incorporation Increases Plant Available Water in A Drastically Disturbed Serpentine Soil. Soil Sci. 2005 , 170 , 939–953. [ Google Scholar ] [ CrossRef ]
- Celik, I.; Ortas, I.; Kilic, S. Effects of compost, mycorrhiza, manure and fertilizer on some physical properties of a chromoxerert soil. Soil Till. Res. 2004 , 78 , 59–67. [ Google Scholar ]
- Cherif, H.; Ayari, F.; Ouzari, H.; Marzorati, M.; Brusetti, L.; Jedidi, N.; Hassen, A.; Daffonchio, D. Effects of municipal solid waste compost, farmyard manure and chemical fertilizers on wheat growth, soil composition and soil bacterial characteristics under Tunisian arid climate. Eur. J. Soil Biol. 2009 , 45 , 138–145. [ Google Scholar ] [ CrossRef ]
- Strauss, P. Runoff, Soil erosion and related physical properties after 7 years of compost application. In Applying Compost-Benefits and Needs, Proceedings of the Seminar 22–23 November 2001, BMLFUW ; Amlinger, F., Nortcliff, S., Weinfurtner, K., Dreher, P., Eds.; European Commission: Vienna, Austria; Brussels, Belgium, 2003; pp. 219–224. [ Google Scholar ]
- Abad, M.; Noguera, P.; Bures, S. National inventory of organic wastes for useas growing media for ornamental potted plant production: Case study Spain. Bioresour. Technol. 2001 , 77 , 197–200. [ Google Scholar ]
- Farrell, M.; Jones, D.L. Food waste composting: Its use as a peat replacement. Waste Manag. 2010 , 30 , 1495–1501. [ Google Scholar ] [ CrossRef ]
- Chong, C. Experiences with Wastes and Composts in Nursery Substrates. HortTechnology 2005 , 15 , 739–747. [ Google Scholar ] [ CrossRef ]
- Raviv, M. Composts in Growing Media: What’s New and What’s Next? Acta Hortic. 2013 , 982 , 39–52. [ Google Scholar ] [ CrossRef ]
- Fornes, F.; Carrión, C.; García-De-La-Fuente, R.; Puchades, R.; Abad, M. Leaching composted lignocellulosic wastes to prepare container media: Feasibility and environmental concerns. J. Environ. Manag. 2010 , 91 , 1747–1755. [ Google Scholar ] [ CrossRef ]
- Santos, A.; Bustamante, M.; Tortosa, G.; Del Moral, R.; Bernal, M. Gaseous emissions and process development during composting of pig slurry: The influence of the proportion of cotton gin waste. J. Clean. Prod. 2016 , 112 , 81–90. [ Google Scholar ] [ CrossRef ]
- Verhagen, J. Stability of growing media from a physical, chemical and biological perspective. Acta Hortic. 2009 , 819 , 135–142. [ Google Scholar ] [ CrossRef ]
- Reinikainen, O.; Herranen, M. Different methods for measuring compoststability and maturity. Acta Hortic. 2001 , 549 , 99–104. [ Google Scholar ]
- Barrett, G.E.; Alexander, P.D.; Robinson, J.S.; Bragg, N.C. Achieving environmentally sustainable growing media for soilless plant cultivation systems—A review. Sci. Hortic. 2016 , 212 , 220–234. [ Google Scholar ]
- Bolechowski, A.; Moral, R.; Bustamante, M.; Bartual, J.; Paredes, C.; Pérez-Murcia, M.; Carbonell-Barrachina, A. Winery–distillery composts as partial substitutes of traditional growing media: Effect on the volatile composition of thyme essential oils. Sci. Hortic. 2015 , 193 , 69–76. [ Google Scholar ] [ CrossRef ]
- Pérez-Murcia, M.D.; Moral, R.; Morenocaselles, J.; Pérez-Espinosa, A.; Paredes, C. Use of composted sewage sludge in growth media for broccoli. Bioresour. Technol. 2006 , 97 , 123–130. [ Google Scholar ] [ CrossRef ]
- Chen, M.; Xu, P.; Zeng, G.; Yang, C.; Huang, D.; Zhang, J. Bioremediation of soils contaminated with polycyclic aromatic hydrocarbons, petroleum, pesticides, chlorophenols and heavy metals by composting: Applications, microbes and future research needs. Biotechnol. Adv. 2015 , 33 , 745–755. [ Google Scholar ] [ CrossRef ]
- Sintim, H.Y.; Bary, A.I.; Hayes, D.G.; Wadsworth, L.C.; Anunciado, M.B.; English, M.E.; Bandopadhyay, S.; Schaeffer, S.M.; Debruyn, J.M.; Miles, C.A.; et al. In situ degradation of biodegradable plastic mulch films in compost and agricultural soils. Sci. Total Environ. 2020 , 727 , 138668. [ Google Scholar ] [ CrossRef ]
- Visconti, D.; Caporale, A.G.; Pontoni, L.; Ventorino, V.; Fagnano, M.; Adamo, P.; Pepe, O.; Woo, S.L.; Fiorentino, N. Securing of an Industrial Soil Using Turfgrass Assisted by Biostimulants and Compost Amendment. Agronomy 2020 , 10 , 1310. [ Google Scholar ] [ CrossRef ]
- Sayara, T.; Sánchez, A. Bioremediation of PAH-Contaminated Soils: Process Enhancement through Composting/Compost. Appl. Sci. 2020 , 10 , 3684. [ Google Scholar ] [ CrossRef ]
- Khan, A.; Kuek, C.; Chaudhry, T.; Khoo, C.; Hayes, W. Role of plants, mycorrhizae and phytochelators in heavy metal contaminated land remediation. Chemosphere 2000 , 41 , 197–207. [ Google Scholar ] [ CrossRef ] [ PubMed ]
- Brown, S.; Christensen, B.; Lombi, E.; McLaughlin, M.; McGrath, S.P.; Colpaert, J.; Vangronsveld, J. An inter-laboratory study to test the ability of amendments to reduce the availability of Cd, Pb, and Zn in situ. Environ. Pollut. 2005 , 138 , 34–45. [ Google Scholar ] [ CrossRef ]
- Chiu, K.K.; Ye, Z.H.; Wong, M.H. Growth of Vetiveria zizanioides and Phragmities australis on Pb/Zn and Cu mine tailings amended with manure compost and sewage sludge: A greenhouse study. Bioresour. Technol. 2006 , 97 , 158–170. [ Google Scholar ]
- de Varennes, A.; Goss, M.J.; Mourato, M. Remediation of a sandy soil contaminated with cadmium, nickel and zinc using an insoluble poiyacrylate polymer. Commun. Soil Sci. Plant Anal. 2006 , 37 , 1639–1649. [ Google Scholar ]
- Angelova, V.R.; Akova, V.I.; Artinova, N.S.; Ivanov, K.I. The effect of organic amendments on soil chemical characteristics. Bulg. J. Agric. Sci. 2013 , 19 , 958–971. [ Google Scholar ]
- Arnesen, A.K.M.; Singh, B.R. Plant uptake and DTPA-extractability of Cd, Cu, Ni and Zn in a Norwegian alum shale soil as affected by previous addition of dairy and pig manures and peat. Can. J. Soil Sci. 1998 , 78 , 531–539. [ Google Scholar ] [ CrossRef ]
- Wong, M.; Lau, W. The effects of applications of phosphate, lime, EDTA, refuse compost and pig manure on the Pb contents of crops. Agric. Wastes 1985 , 12 , 61–75. [ Google Scholar ] [ CrossRef ]
- Ye, Z.; Wong, J.; Wong, M.; Lan, C.; Baker, A. Lime and pig manure as ameliorants for revegetating lead/zinc mine tailings: A greenhouse study. Bioresour. Technol. 1999 , 69 , 35–43. [ Google Scholar ] [ CrossRef ]
- Silva, M.T.B.; Moldes, A.B.; Seijo, Y.C.; Viqueira, F.D.-F. Assessment of municipal solid waste compost quality using standardized methods before preparation of plant growth media. Waste Manag. Res. 2007 , 25 , 99–108. [ Google Scholar ] [ CrossRef ] [ PubMed ]
- Parchomenko, A.; Borsky, S. Identifying phosphorus hot spots: A spatial analysis of the phosphorus balance as a result of manure application. J. Environ. Manag. 2018 , 214 , 137–148. [ Google Scholar ] [ CrossRef ] [ Green Version ]
- Tognetti, C.; Mazzarino, M.; Laos, F. Improving the quality of municipal organic waste compost. Bioresour. Technol. 2007 , 98 , 1067–1076. [ Google Scholar ] [ CrossRef ] [ PubMed ]
- Adamcová, D.; Radziemska, M.; Ridošková, A.; Bartoň, S.; Pelcová, P.; Elbl, J.; Kynický, J.; Brtnický, M.; Vaverková, M.D. Environmental assessment of the effects of a municipal landfill on the content and distribution of heavy metals in Tanacetum vulgare L. Chemosphere 2017 , 185 , 1011–1018. [ Google Scholar ] [ CrossRef ]
- Carballo, T.; Gil, M.; Calvo, L.F.; Morán, A. The Influence of Aeration System, Temperature and Compost Origin on the Phytotoxicity of Compost Tea. Compos. Sci. Util. 2009 , 17 , 127–139. [ Google Scholar ] [ CrossRef ]
- Pérez-Gimeno, A.; Navarro-Pedreño, J.; Almendro-Candel, M.B.; Gómez, I.; Jordan, M. Environmental consequences of the use of sewage sludge compost and limestone outcrop residue for soil restoration: Salinity and trace elements pollution. J. Soils Sedim. 2015 , 16 , 1012–1021. [ Google Scholar ] [ CrossRef ]
- Fagnano, M.; Adamo, P.; Zampella, M.; Fiorentino, N. Environmental and agronomic impact of fertilization with composted organic fraction from municipal solid waste: A case study in the region of Naples, Italy. Agric. Ecosyst. Environ. 2011 , 141 , 100–107. [ Google Scholar ] [ CrossRef ]
- Smith, S.R. A critical review of the bioavailability and impacts of heavy metals in municipal solid waste composts compared to sewage sludge. Environ. Int. 2009 , 35 , 142–156. [ Google Scholar ] [ CrossRef ]
- Page, K.; Harbottle, M.; Cleall, P.; Hutchings, T. Heavy metal leaching and environmental risk from the use of compost-like output as an energy crop growth substrate. Sci. Total Environ. 2014 , 487 , 260–271. [ Google Scholar ] [ CrossRef ] [ Green Version ]
- Sileshi, G.W.; Jama, B.; Vanlauwe, B.; Negassa, W.; Harawa, R.; Kiwia, A.; Kimani, D. Nutrient use efficiency and crop yield response to the combined application of cattle manure and inorganic fertilizer in sub-Saharan Africa. Nutr. Cycl. Agroecosyst. 2019 , 113 , 181–199. [ Google Scholar ] [ CrossRef ]
- Sokka, L.; Antikainen, R.; Kauppi, P. Flows of nitrogen and phosphorus in municipal waste: A substance flow analysis in Finland. Prog. Ind. Ecol. Int. J. 2004 , 1 , 165. [ Google Scholar ] [ CrossRef ] [ Green Version ]
- Guo, Z.; Zhang, J.; Fan, J.; Yang, X.; Yi, Y.; Han, X.; Wang, D.; Zhu, P.; Peng, X. Does animal manure application improve soil aggregation? Insights from nine long-term fertilization experiments. Sci. Total Environ. 2019 , 660 , 1029–1037. [ Google Scholar ] [ CrossRef ] [ PubMed ]
- Wei, Y.; Li, J.; Shi, D.; Liu, G.; Zhao, Y.; Shimaoka, T. Environmental challenges impeding the composting of biodegradable municipal solid waste: A critical review. Resour. Conserv. Recycl. 2017 , 122 , 51–65. [ Google Scholar ] [ CrossRef ] [ Green Version ]
- Martínez-Blanco, J.; Colón, J.; Gabarrell, X.; Font, X.; Sánchez, A.; Artola, A.; Rieradevall, J. The use of life cycle assessment for the comparison of biowaste composting at home and full scale. Waste Manag. 2010 , 30 , 983–994. [ Google Scholar ] [ CrossRef ] [ Green Version ]
- Colón, J.; Cadena, E.; Pognani, M.; Barrena, R.; Sánchez, A.; Font, X.; Artola, A. Determination of the energy and environmental burdens associated with the biological treatment of source-separated Municipal Solid Wastes. Energy Environ. Sci. 2012 , 5 , 5731–5741. [ Google Scholar ] [ CrossRef ] [ Green Version ]
- Quirós, R.; Villalba, G.; Muñoz, P.; Colón, J.; Font, X.; Gabarrell, X. Environmental assessment of two home composts with high and low gaseous emissions of the composting process. Resour. Conserv. Recycl. 2014 , 90 , 9–20. [ Google Scholar ] [ CrossRef ]
Click here to enlarge figure
Compost Feedstock | Experimental Conditions | Effect | Ref. |
---|---|---|---|
Green wastes | Compost was applied at three different rates: 5 kg/each tree, 10 kg/each tree and 15 kg/each tree | Compost improved soil organic matter, available phosphorus and available potassium content. The high-level application amount (15 kg/tree) had the greatest effect on soil improvement. Under this rate and compared with the control treatment, soil pH decreased to 7.28–7.45, soil organic matter content reached more than 35 g·kg . Soil total nitrogen, soil available phosphorus and soil available potassium increased by 25–28%, 200–400% and 80–177%, respectively. Additionally, the soil microbial structure was changed such that bacterial abundance increased by 12–13%. | [ ] |
Mix of food wastes, animal bedding and manure | Compost was incorporated in soil for 12 years (33% by volume) with the use of a backhoe, and annual top dressing with mulch | Soils exhibit improved (reduced) bulk density, increased active carbon and increased potentially mineralizable nitrogen. Compared to unamended soils, improvements were found in aggregate stability (72.41%), available water-holding capacity (0.22%), total organic matter (8.43%), potentially mineralizable nitrogen (27.53 mg/kg), active carbon (1022.47 mg/kg) and reduction in bulk density (0.89 g/cm ). | [ ] |
Sheep manure and wheat straw | Continuous application of compost for 5 years in the proportion of 60:40 (volume basis) | Higher productivity of Prunus salicina (21.4%), greater fruit diameter (7.8%) and heavier fruit weight (22.4%) compared to unamended soil. Additionally, the amended soil by compost increased the SOM and water-soluble C fraction in parallel with an increase in microbial parameters (microbial biomass C, adenosine triphosphate (ATP), basal respiration and dehydrogenase). | [ ] |
Mixed source and yard waste | Five years’ incorporation of compost (40%, v/v) with soil | Compost incorporation increased hydraulic conductivity by a factor of 22, but the incorporated yard waste compost treatment tended to show a faster reduction in hydraulic conductivity over time (5 years) than the mixed compost. | [ ] |
Digestates and compost | Digestate and compost were applied at a rate of 100 m /ha for four years | Increased pH of the soil and improved the biological soil activity (e.g., enzymatic activities). | [ ] |
Dairy waste | Dairy waste compost was applied at a rate of 100 T/ha for 5 years | The dairy waste compost increased organic carbon by 143 and 54% compared to ammonium sulfate and liquid dairy waste treatments, respectively, applied at the same available N level (200 kg N/ha), whereas the C pool was enhanced by 115%. | [ ] |
Cattle manure | Compost was applied annually for 5 years | Organic C and total nitrogen concentrations were increased up to 2.02t C/ha. yr and 0.24t N/ha. yr. | [ ] |
Yard waste | Compost was incorporated into soil (21%, v/v) | A two-fold increase in plant water availability and an increase in the ability of the plants to access water resources through root proliferation. | [ ] |
Manure organic wastes | Compost and manure were used at 25 t/ha for a 5-year field experiment with a semi-arid Mediterranean soil | Compost and manure treatments increased available water content (AWC) of soils by 86 and 56%, respectively, as a result of the increase in micro- and macro-porosity. However, total porosity and saturated hydraulic conductivity were highest under the compost treatment. | [ ] |
Municipal solid waste | Municipal solid waste compost applied annually over 5 years at a rate of 80 t/ha | Wheat grain yield was enhanced on average by 246% compared to the control. | [ ] |
Different organic wastes | For five years, compost applied annually at an amount of between 30 and 50 m in plots of 25 × 12 m | Compost application resulted in 67% reduced soil erosion, 60% reduced run-off, 8% lower bulk density and 21% higher organic matter (OM) content compared to control plots. | [ ] |
MDPI stays neutral with regard to jurisdictional claims in published maps and institutional affiliations. |
Share and Cite
Sayara, T.; Basheer-Salimia, R.; Hawamde, F.; Sánchez, A. Recycling of Organic Wastes through Composting: Process Performance and Compost Application in Agriculture. Agronomy 2020 , 10 , 1838. https://doi.org/10.3390/agronomy10111838
Sayara T, Basheer-Salimia R, Hawamde F, Sánchez A. Recycling of Organic Wastes through Composting: Process Performance and Compost Application in Agriculture. Agronomy . 2020; 10(11):1838. https://doi.org/10.3390/agronomy10111838
Sayara, Tahseen, Rezq Basheer-Salimia, Fatina Hawamde, and Antoni Sánchez. 2020. "Recycling of Organic Wastes through Composting: Process Performance and Compost Application in Agriculture" Agronomy 10, no. 11: 1838. https://doi.org/10.3390/agronomy10111838
Article Metrics
Article access statistics, further information, mdpi initiatives, follow mdpi.
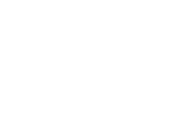
Subscribe to receive issue release notifications and newsletters from MDPI journals
Compost: Production, Quality, and Use in Commercial Agriculture
- November 2010

- University of Idaho
Abstract and Figures

Discover the world's research
- 25+ million members
- 160+ million publication pages
- 2.3+ billion citations

- Dwi Probowati Sulistyani

- R B Heryanto

- Akhilesh Kumar Pandey
- Mamdouh Serag
- Abd El-Hamid Khedr
- Reham El-Barougi
- Hanan Abu El-Agras

- Afolabi Ayomide Emmanuel
- Irenosen Addeh

- Meire Cristina Andrade Cassimiro da Silva

- M. van de Kamp
- G. B. Willson

- Gene Logsdon
- Nyle C. Brady
- M Debertoldi
- Iw Debertoldi
- Daniel Dreyfus
- Ray Alleman
- Dennis Demmel
- M Trillus-Gray
- Recruit researchers
- Join for free
- Login Email Tip: Most researchers use their institutional email address as their ResearchGate login Password Forgot password? Keep me logged in Log in or Continue with Google Welcome back! Please log in. Email · Hint Tip: Most researchers use their institutional email address as their ResearchGate login Password Forgot password? Keep me logged in Log in or Continue with Google No account? Sign up
Lomi review: Kitchen appliance transforms food waste into compost
We love the made-in-Canada countertop composting appliance
You can save this article by registering for free here . Or sign-in if you have an account.
Article content
Reviews and recommendations are unbiased and products are independently selected. Postmedia may earn an affiliate commission from purchases made through links on this page.
Lomi is made in Canada (Kelowna, B.C. to be specific) but is loved beyond our borders as a wonderfully efficient way to reduce organic kitchen waste volume (think carrot peelings, eggshells, soggy vegetable clippings, leftovers and coffee grounds) by up to 80 per cent.
Enjoy the latest local, national and international news.
- Exclusive articles by Conrad Black, Barbara Kay and others. Plus, special edition NP Platformed and First Reading newsletters and virtual events.
- Unlimited online access to National Post and 15 news sites with one account.
- National Post ePaper, an electronic replica of the print edition to view on any device, share and comment on.
- Daily puzzles including the New York Times Crossword.
- Support local journalism.
Create an account or sign in to continue with your reading experience.
- Access articles from across Canada with one account.
- Share your thoughts and join the conversation in the comments.
- Enjoy additional articles per month.
- Get email updates from your favourite authors.
Don't have an account? Create Account
The popular kitchen appliance takes the ick out of composting by transforming kitchen waste into garden-quality fertilizer for indoor or outdoor plants. I’ve tested the Lomi and here are my thoughts.
Summary : Lomi is a Canadian success story and an eco-friendly and easy way to deal with the gross task of composting and adding some dirt to our garden. I tested the original Lomi 1 model, but the newer Lomi 2 is available for $599 USD.
Price : $685 Where to buy : Amazon
You might be interested in the Lomi if;
- You hate collecting the compost because it gets stinky, slimy and gross
- You don’t fancy the flies that sometimes find a way into the compost bin under your sink
- You don’t want to go back to getting-the-worms-to-do-the-dirty-work kind of composting
- You want to lower your carbon footprint by being less of a debaucherous human
- When you say you want to contribute something to Mother Earth, you don’t mean copious amounts of trash
- You like funky technology that makes your little home just a little more eco-friendly
In the box
- Activated carbon for two different filters on the machine to help snuff the stink
- A pack of 45 LomiPods which are “a proprietary blend of probiotics that improve the speed of degradation the reduction of smell and most importantly help create the most healthy end product to add to gardens/lawn planters”
- A paper instruction manual means you don’t have to consult a website or video to get started. This is very much appreciated by the team here at Shopping Essentials
Product reviews, deals, roundups and interviews to help you make better buying decisions.
- There was an error, please provide a valid email address.
By signing up you consent to receive the above newsletter from Postmedia Network Inc.
A welcome email is on its way. If you don't see it, please check your junk folder.
The next issue of Shopping Essentials will soon be in your inbox.
We encountered an issue signing you up. Please try again
The cardboard packaging in the box is, you guessed it, compostable if you break it down into smaller pieces and introduce it slowly, as in about 10 per cent of the total compost at a time.
What it didn’t come with? Enough composting material for me to run the Lomi through a few rounds. My neighbours were more than happy to oblige by dropping off their kitchen scraps and were fully impressed by how the fancy bit of technology took a full bucket of Lomi-approved waste (more on that below) and turned it into something far less offensive.
What does Lomi do?
The countertop composter that does an ugly job but looks pretty good while doing it — it’s a sleek white-ish matte machine — breaks down organic waste into fragments that can be mixed with existing compost, added to your green bin with other compostable materials or if you use the right mode on your Lomi, used as soil that is packed with nutrients and organic content.
Setting it up
It’s not hard. The most challenging part might be finding a spot for your Lomi. Depending on how often you use it, you could keep it in a pantry and pull it out once a week to do its job. Mine fits on my counter and although it is pretty enough, I really don’t fancy hogging counter space when not in use. I’d like to save that for more important things like dirty dishes and meal prep.
Some specs :
- Lomi is 22 lbs with an off-white, matte-finish
- It is 16 x 12 inches
- Three processing cycles; drying, mixing and cooling
- You can hear it operating, but it’s like a low hum, and we kind of like the sound of work being done, especially when it’s not us doing the work
- Two filters require carbon pellets (that’s our fancy word) which need to be replaced once, maybe twice a year
More about the processing modes
These vary based on how long you want to wait and what final result you want.
Eco-express — Three to five hours. Offers quickest results with low energy consumption.
Lomi approved — Five to eight hours. This mode is for bioplastics , compostable commercial goods and packaging that is Lomi-approved. You can find more on their website .
Grow — 16 to 20 hours. When you are operating in this mode, it runs at low heat “to preserve the microorganisms and bacteria most helpful to soil.”
What Lomi likes to be fed and what you ought to trash
It likes a diverse diet, so a mixed assortment works well and gives the best results.
You can add everything from fruits and vegetables to leftovers, plate scrapings, meat scraps, soft bones and shells, coffee filters and grounds, tea bags, nuts, bread, pasta, rice, cheese yogurt, plants, flowers and yard trimmings.
Do not add hard animal bones, cooking oils, fruit pits, soiled diapers, cigarettes, metal, plastic, glass, foil wrap, styrofoam or pet waste.
My experience
I filled the bucket to the maximum line, added one of the LomiPods and closed the lid on the stink. When I hit start, I didn’t realize it defaults to Eco-Express mode when what I really wanted was a little something something for my hungry houseplants. That’s fine, I had to try every mode anyhow but just a word of caution.
By the way, the bucket where you chuck it all is made from aluminum with a non-stick coating and is dishwasher safe.
It hums along, not quite as loudly as my dishwasher or microwave and quietly enough you can listen to music or have a Zoom meeting nearby. A few times, it gurgles and grumbles as it digests the contents but when it’s done, it’s a sight to behold.
In Eco-Express mode, I started with a big mess of egg shells, cooked quinoa, half a grapefruit, vegetable peels and pieces and some fruit that was well past its prime time.
When I excitedly opened the lid several hours later, there was what I would describe as a small amount of mulch-like sawdust at the bottom of the once two-thirds full bucket. Dry, clean and virtually scent-free.
The grow mode delivers something softer and richer and ready to help my houseplants become even more luscious and lovely. It’s best to do this one overnight because it is the longest mode. The Lomi-approved mode can process bioplastics (the site has more details) like Uber Eats containers, forks and knives.
For those concerned about how disruptive running a Lomi might be, the noise level is less than 60 decibels For reference, a normal conversation is about 60 decibels and a lawn mower is about 90 decibels.
It’s easy to clean by wiping the exterior with a soapy cloth and the bucket is dishwasher safe.
Others’ take on the Lomi
Reviews are by and large fantastic. The makers say they have sold more than 85,000 units, sharing plenty of customer reviews, here . One happy purchaser calls it “their best friend,” which we think may be a bit much but other than that, we kind of agree on all the applause.
We did find one critic scoffing at the technology saying it is counterintuitive given it takes hours of electricity to do its job. But (we did the math) and using anywhere from .6 kWh to one kWh, (that’s during each hour of its longest mode) is hardly significant given the average Canadian uses about 1,000 kWh a month .
A bit about Lomi
The minds behind the Lomi began in 2017 with the Pela Case , the first biodegradable phone case. In April 2021 the Lomi took off. Khloe Kardashian, out of the blue, (ie, she wasn’t sent a sample to review) dubbed it the Gift of the Year , says Gareth Everard, Lomi’s chief marketing officer.
Last year the Kelowna-based company which created the kitchen gadget after tonnes of research and development done by hardware engineers, software experts and soil scientists, pre-sold nearly 50,000 units, mostly in Canada and the U.S., the bulk bought by Americans.
Why is it called Lomi? It is cute, approachable and evocative of EVE from the film WALL-E . It also plays the words ‘ loam ’ and ‘soil.’
Why is it so popular? “I think people really like the reduction in mess and the no stink,” Everard says. “The thing that is really redeeming and heartwarming is how much impact people are saying the Lomi has on the volume of garbage. They reduce the number of bags they are bringing out to the curb.”
Where to buy : Amazon
Shopping Essentials is a category written by research-obsessed shopping experts. Explore product reviews, recommendations and launches — plus behind-the-scenes info on your favourite brands and hidden gems — learn more here or sign up for our newsletter .
Postmedia is committed to maintaining a lively but civil forum for discussion. Please keep comments relevant and respectful. Comments may take up to an hour to appear on the site. You will receive an email if there is a reply to your comment, an update to a thread you follow or if a user you follow comments. Visit our Community Guidelines for more information.
Driver charged with killing Johnny Gaudreau and his brother cries in court after he's denied bail
Quebec wants anti-islamophobia adviser to resign after she calls for hiring more muslim professors, jordan peterson: i am ready for my re-education. who will be my tutor, criminal trial for freedom convoy organizers lich and barber comes to an end after one year, woman, 36, dies after she was denied liver transplant over alcohol use. should health-care be conditional, more than half of pet owners say pet guilt is the real deal.
The right way to buy your way out of the dog house
Canada Goose Glacier Trail Sneaker review: Classic rugged luxury
A waterproof shoe with a fierce silhouette
Advertisement 2 Story continues below This advertisement has not loaded yet, but your article continues below.
No-makeup makeup: How to get the look
From skincare to makeup, we're rounding up everything you need
The best Amazon deals in Canada right now
Take advantage of these Amazon Canada deals before they're gone
Editor favourites: Our top finds this month
Products we couldn’t get enough of this August
This website uses cookies to personalize your content (including ads), and allows us to analyze our traffic. Read more about cookies here . By continuing to use our site, you agree to our Terms of Service and Privacy Policy .
You've reached the 20 article limit.
You can manage saved articles in your account.
and save up to 100 articles!
Looks like you've reached your saved article limit!
You can manage your saved articles in your account and clicking the X located at the bottom right of the article.

COMMENTS
Abstract. This review analyses the main challenges of the process of food waste composting and examines the crucial aspects related to the quality of the produced compost. Although recent advances have been made in crucial aspects of the process, such composting microbiology, improvements are needed in process monitoring.
To make better use of kitchen waste compost, 45-mm particle size screening is suggested at an industrial-scale composting plant. ... competing financial interests or personal relationships that could have appeared to influence the work reported in this paper. Acknowledgements. This research was supported by the major science and technology ...
Improper kitchen and agricultural waste management causes substantial health and environmental problems. The microbiological aspects of compost are crucial in optimizing its functionality as an organic fertilizer. This systematic review studies the relationship between kitchen and agricultural waste composts and their bacterial communities as well as the physicochemical properties. A total of ...
KITCHEN WASTE COMPOSTING: A SUSTAINABLE WASTE MANAGEMENT TECHNIQUE. N. Shukla, S. K. Juneja. Published 2016. Environmental Science. Population explosion, rapid industrialization and trend of urbanization have resulted in massive migration of people from rural to urban areas. As a result, thousands of tons of waste are being generated.
Kitchen waste is known for having high oil, fat, water, organic matter and salt, and contains many trace elements that are prone to corruption and bacterial growth (Komilis & Ham, 2006). Therefore, improper treatment will cause secondary pollution to the environment. Composting technology is an effective means to reduce environmental pollution.
Purpose This study was carried out to compare the effects of composted kitchen waste (KW) and poultry manure (PM) soil amendments on growth, yield and heavy metal uptake in edible leaf of Corchorus olitorius. Methods Kitchen waste and PM composted for 8 weeks were applied as soil amendment at the rate of 0 (no amendment), 5, 10 and 15 t ha−1. Corchorus seeds were sown 2 weeks after ...
According to Haydar and Mahood, [9] compost from kitchen waste has higher carbon to nitrogen content as compared to compost processed from solid waste produced in urban centres. This means compost ...
Here is a practical case of kitchen waste composting processor. Based on the principle of DANO roller, Guangdong Modern Agricultural Equipment Research institute independently developed the non-power composting tank for kitchen waste, and then improved it into the air-box composting machine for kitchen waste . The main structure includes ...
The addition of compost increased the organic matter (1.21% to 10.27%) and moisture content (4.23% to 5.88%) however no major change was observed in pH (8.04 to 8.4). The results showed that the ...
Kitchen waste (KW) production has risen considerably due to the increasing affluence of populations and a booming catering sector. If not properly disposed, KW can bring serious issues for the environment. Composting is widely used as an efficient method for the resource utilization of KW. In the present paper, the effects of different ratios of ingredients (corn straw: garden waste = 4:1 ...
With increases in global population and urbanization, the production of Municipal Solid Waste (MSW) is growing rapidly, thus contributing to social and environmental concerns for sustainable waste management. This study addresses the research gap in optimizing composting, hypothesizing that integrating best practices and recent innovations can enhance the efficiency of the process. Data were ...
Management of kitchen waste reduces or eliminates adverse impacts on land, contamination of the atmosphere, soil and water. The aim of the present study is to convert kitchen waste into useful product for better growth and quality of crops so as to promote sustainable waste management. Keywords: Municipal solid waste, kitchen waste, composting.
Composting is the controlled conversion of degradable organic products and wastes into stable products with the aid of microorganisms. Composting is a long-used technology, though it has some shortcomings that have reduced its extensive usage and efficiency. The shortcomings include pathogen detection, low nutrient status, long duration of composting, long mineralization duration, and odor ...
PDF | Composting has become a preferable option to treat organic wastes to obtain a final stable sanitized product that can be used as an organic... | Find, read and cite all the research you need ...
Background Composting is one of the integrated waste management strategies used for the recycling of organic wastes into a useful product. Composting methods vary in duration of decomposition and potency of stability, maturity and sanitation. This study was aimed to investigate the comparative effectiveness of four different methods of composting viz. windrow composting (WC), Vermicomposting ...
Composting can divert organic waste from landfills, reduce landfill methane emissions, and recycle nutrients back to soils. However, the composting process is also a source of greenhouse gas and air pollutant emissions. Researchers, regulators, and policy decision-makers all rely on emissions estimates to develop local emissions inventories and weigh competing waste diversion options, yet ...
The Defence Colony in the state of Delhi is practising composting for its kitchen waste using effective microorganisms (EM1) microbial solution-based pit composting. ... 11.20% paper, 2.10% inerts, and the remaining 11% including wood, metal, glass, and other parts ... (Hoornweg et al. 2000 and Waste to Energy Research Council 2012).
This guide will help you turn your food waste into beautiful earthy compost in five simple steps. 1. Select your food scraps. Start with fruits and veggies — the skin of a sweet potato, the top ...
The paper is about processing daily Food Waste in such a way that we will get Fertilizer as our product. In this process ... International Journal of Trend in Research and Development, Volume 5(3), ISSN: 2394-9333 ... Development and Evaluation of a Small Scale Kitchen Waste Composting Machine,,"IOSR Journal of Engineering (IOSRJEN_, vol. 04 ...
Composting has become a preferable option to treat organic wastes to obtain a final stable sanitized product that can be used as an organic amendment. From home composting to big municipal waste treatment plants, composting is one of the few technologies that can be practically implemented at any scale. This review explores some of the essential issues in the field of composting/compost ...
Here, it is demonstrated that the use of 0.028 M glucose and an appropriate source of N2-fixing bacteria (green waste-derived compost) can result in increased grass dry matter yields of over 50% ...
The design and operation of waste-to-energy technology are reliant on process variable modelling and optimization. A few research have been looking at the application of AI to estimate the process variables of MSW management. The genetic algorithm was used to predict nitrogen losses and maturity during composting of waste materials.
Five of these states enacted composting requirements over this span, allowing the scientists to compare numbers before and after. Only Massachusetts, which implemented a food waste law in 2014, made a substantial reduction, lowering the amount by 13%. Researchers partially credited the state's simple, easy-to-follow system with its success.
Last year the Kelowna-based company which created the kitchen gadget after tonnes of research and development done by hardware engineers, software experts and soil scientists, pre-sold nearly ...
Composting is another reliable waste disposal method, particularly for domestic waste that is considered organic. Thus, composting stands as the most available opportunity to recover material from the organic component of municipal solid waste as it can be reused in the form of a biofertilizer to enhance soil fertility (Cesaro et al., 2015).